A sustainable university is defined by Velazquez et al. as ‘‘A higher educational institution, as a whole or as a part, that addresses, involves and promotes, on a regional or a global level, the minimization of negative environmental, economic, societal, and health effects generated in the use of their resources to fulfill its functions of teaching, research, outreach and partnership, and stewardship in ways to help society make the transition to sustainable lifestyles.’’ In this article, we will understand how a campus can go in the direction of sustainable development in regards to energy consumption (Alshuwaikhat and Abubakar).
Why a University Campus?
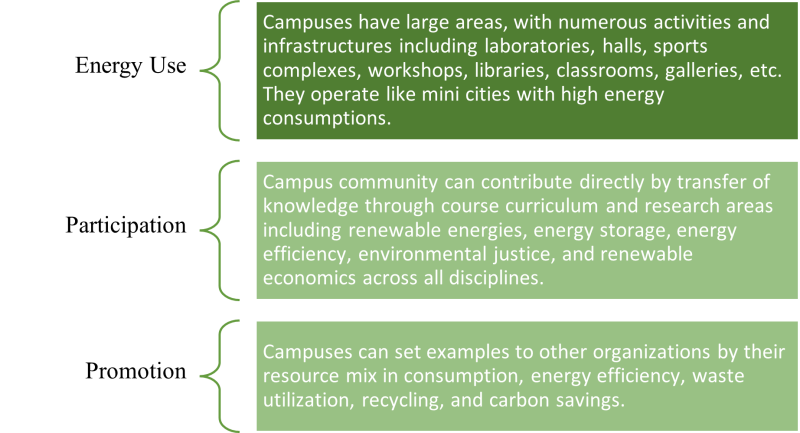
Steps in the Direction of Green Power
Scoping of Resources
The utilization of green energy is location specific. The system design for renewable resources depends on the abundance of resources in the chosen area. This is demonstrated in how universities in Western Australia have abundant solar energy while universities in Scotland are windy, which drives turbines for electricity.
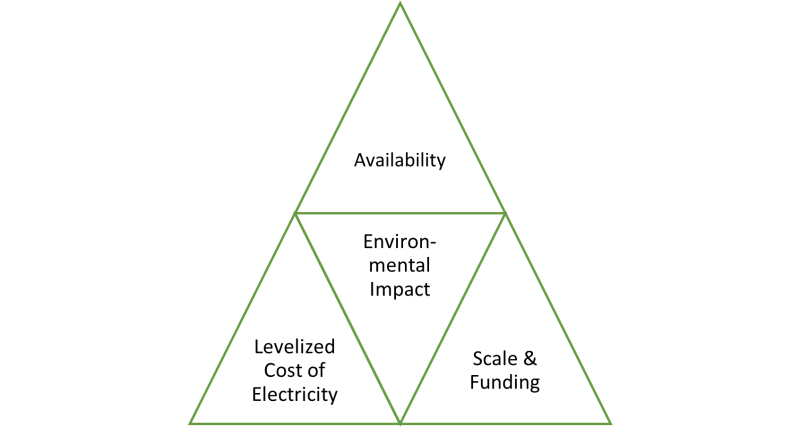
- Availability of Resource: This evaluation is based on location-specific details for understanding the abundance of renewable resources. This includescwind-speed maps for wind energy, solar irradiance maps for solar energy, subsurface temperature logs for geothermal energy, flow rate and head for hydropower, wave height and tidal range for ocean energies, and organic waste generation for biomass/biofuels.
- Funding: Renewable energy agencies have accessible funds to assist the shift from a fossil to green power-based economy. Such funds are available to resource and scale specific investment commitments by clean energy initiatives. The total investment commitment by Australia’s Clean Energy Finance Corporation for solar resources is over $1 billion, and for wind resources is nearly $720 million (Australian Renewable Energy Agency).
- Levelized cost of electricity: Technology developments like high-efficiency solar panels, smart rotors, floating turbines, improved forecasting for grid reliability and resilience, etc. are reducing the cost of electricity generation by renewable resources and thereby promoting the transition. The most cost-effective resources are ranked higher in this scoping analysis by the International Energy Agency.
- Environmental Impact: The aim of green energy is to reduce carbon emissions. Approximate life-cycle carbon emissions with social and ecological barriers are accounted in ranking the environmental benefits of the resources.
- Scale: The scale of development depends on the demand analysis and area required by the resource for exploitation. This is often based on the stipulated size of the renewable installation vis-à-vis the requirement. The installation size is governed by the effective cost benefit from the project too; for instance a small hydropower wind project wouldn’t be as economical as a large-scale installation in the project lifetime (US Office of Energy Efficiency & Renewable Energy).
Demand Analysis
The next step in green power development is demand analysis, i.e., the consumption data on a daily, monthly, and yearly basis. It is required to comprehend the maximum power to be delivered by renewable systems. And as campuses have to supply not only the power, but also the heating and cooling requirements in the buildings, these resources need to be exploited both for electricity and thermal energy. It is also important to know the power distribution aerially across the campus as electricity generated in one part of the campus (example: parking spaces) is transmitted to the demand areas (example: laboratories) (The University of Western Australia).
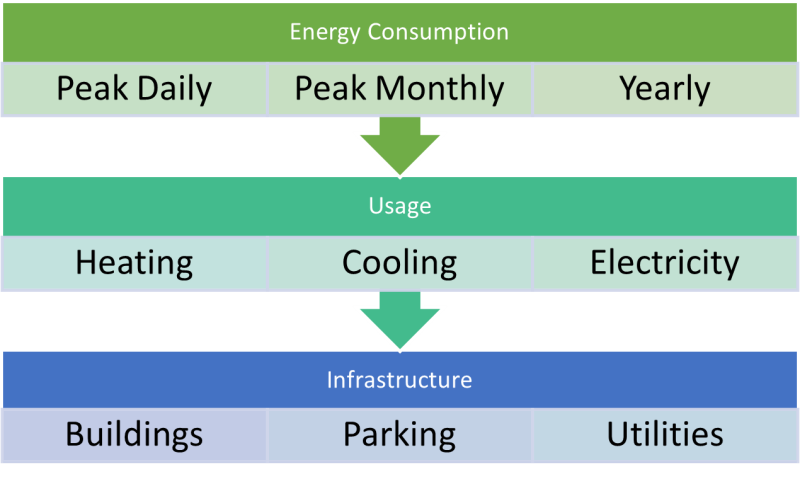
System Design and Performance Analysis
A renewable system is designed for self-sufficiency in light of peak-load management and grid stability. System design is a complex process with many permutations and combinations in terms of component options, storage solutions, and plant layout choices for efficient and sustainable power generation. The design process is as follows:
- Components of the renewable system: For solar, photovoltaics (PV) modules/solar thermal, inverters, and transformer options exist in the market. For wind, turbine types (rotor diameter, hub height) and configuration possibilities are plentiful. For biomass, feedstocks, gasifier, and generator selection are varied.. These selections are generally based on project types, compact sizes, high efficiency, and low installation costs (Albadia et al.).
- Energy Storage: The energy production varies seasonally/daily as per flowing currents of renewable energies ( solar irradiance, wind speeds, wave heights). Therefore, the excess generated in peak hours needs to be stored for future utilization, such as in times of nonavailability of the resources. This governs the system’s performance. The system performance is assessed by the energy flexibility and security supplied by the hybrid energy mix. A consistent energy resource/storage integrated with a variable resource such as a combination of biomass, solar, hydro, wind, geothermal, or wave energy leads to the enhanced reliability of the overall system and an optimum energy balance in peak-load scenarios. The system performance can be further boosted by metering the excess to the grid, supplying additional energy during peak demand. This metering system requires maintenance of grid stability.
- Layout: Utilization of available campus areas for energy generation is the best go-to strategy. Solar PV on roofs and parking spaces, solar trees in parks and gardens, solar street lighting, rooftop wind turbines, windmills in open ground on campus, small-scale biomass gasifiers near food courts for utilization of organic wastes, and hydraulic turbines near surface runoffs/small rivers near campus are some examples of campus space energy generation (Alshuwaikhat and Abubakar).
Cost-Benefit Analysis and Carbon Savings
The preliminary cost-benefit analysis can be carried out using basic tools like net present value, discounted payback period, and levelized cost of electricity (LCOE). The capital expenditure should consider renewable energy system components and energy storage devices. There will be additional operating expenditures throughout the entire life of the system for maintenance and operations. Revenues will have cost savings calculated based on the discounted rates as per renewable energy system guidelines and the cost of electricity from the grid (this electricity will instead be generated by the renewable system on the campus). The system results are profitable if the LCOE of the renewable energy system estimates to be lower than the LCOE of the fossil-fuel-based system. The system can further benefit from the exemption of emission taxes and funding obtained under clean energy initiatives of various countries (Asad et al.).
The carbon-emission savings by transitioning from fossil-fuel energy production to renewable energy systems evaluates the carbon footprint throughout the generation life cycle. On average, renewable energy systems have a carbon saving of approximately 75% over fossil-fuel-based systems. The university can participate in government’s carbon-crediting scheme and earn carbon credit units per ton of saved CO2 emissions. Universities can later sell these credits to the government or businesses and generate supplementary incomes (Australian Government).
The Way Ahead
Each one of us is part of the global campus communities as a student, an alumnus, a researcher, or an academic. It is only right for us to understand and propagate the values of environmental sustainability and green campus initiatives. Such implementations can have consequences that go beyond the university campus: spillover effects take place in the totality of the region hosting the university. Thus, in the words of Emily Dickinson, “One step at a time is all it takes to get there.”