Energy systems models such as those analyzed by the UN Intergovernmental Panel on Climate Change (IPCC) have suggested that CO2 storage must achieve rates of 10 Gt of CO2 per year by 2050 in nearly all technological pathways to reach net-zero of the global economy (Fig. 1). This is the key driver for the large-scale deployment of CCS technology. As a result, the implied energy infrastructure must reach a scale nearly matching that of the current global oil and gas infrastructure.
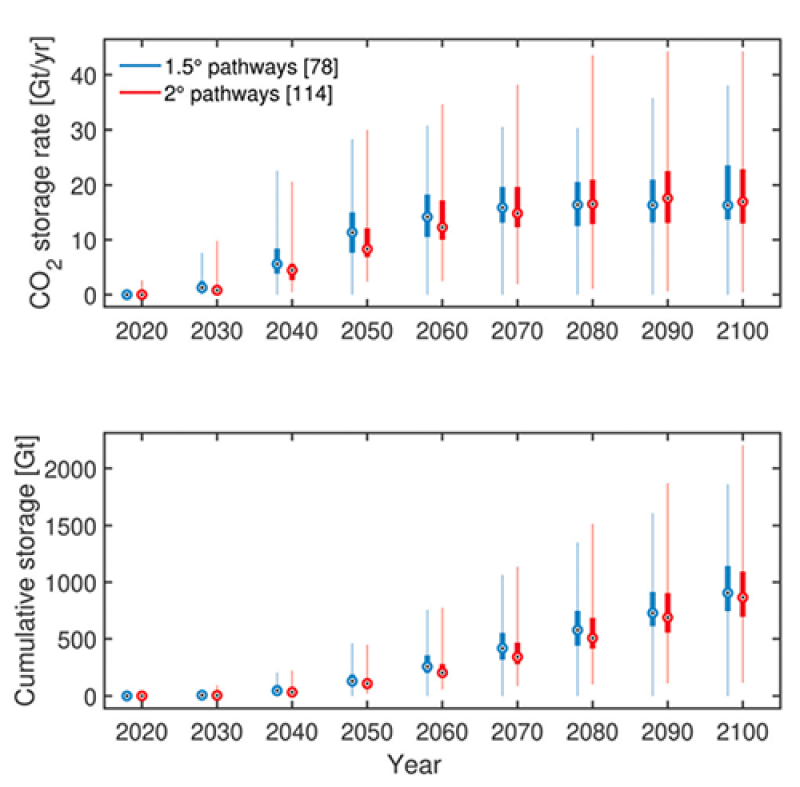
Globally, cumulative CO2 storage targets by 2100 have been identified by the IPCC to range between 348 Gt to up to 1218 Gt. Regionally, the European Union and the United Kingdom have a commensurate scale of carbon storage in their climate-change-mitigation plans including storage-rate scenarios with combined over 500 Mt CO2 injected underground per year by 2050.
Overview of Current Status of CCS Technology
To evaluate the plausibility of the Gt-scale deployment of CCS, components surrounding the status of CCS provide some initial insights. First, there are operational CCS facilities around the world that are demonstrating CO2 storage can be performed safely at industrial rates and that global deployment of CCS has been growing at a constant annual rate of 8.6% (Zahasky & Krevor, 2020). Within Europe, there are two operational CCS facilities in Norway (Sleipner and Snøhvit) and one in Croatia; these three projects have a combined injection capacity of 1.7 Mt yr-1. Secondly, a recent comparison shows that from an engineering perspective, the suggested global scaleup of CO2 storage by midcentury is feasible by converting the historical rates of hydrocarbon wells drilled offshore of the US and Norway into cumulative CO2 storage. Finally, existing estimates of global storage resources suggest that there are vast volumes of pore space underground suitable for sequestering CO2. Recent evaluations identify a storage resource base between 10,000–30,000 Gt available worldwide. Regionally, the combined estimate of resources in Europe is 259 Gt, including resources distributed among EU member states (88 Gt), and offshore UK (78 Gt) and Norway (94 Gt).
Gaps in Existing Energy Systems Models
There are, however, gaps in the representation of realistic development trajectories of subsurface storage resource use in current energy system models. As a result, the amount of CO2 stored in these models is unconstrained by factors that might limit the injection rates and their scale-up potential including political, financial, social, and geophysical limitations. As stated, geologically based storage resource assessments are widely available and have been carefully assessed; however, these estimates still contain significant uncertainty that ranges between one to two orders of magnitude (Fig 2). Therefore, understanding the geological features alone cannot be used to describe patterns of depletable natural resource use including subsurface storage resources for CO2. Our current position in the state of knowledge for CO2 storage is similar to the understanding of global oil resources in the 1950s. Accurate predictions of the resource base for oil production only became available as oil was produced across thousands of projects around the globe. While this is likely to be the case for CO2 storage, currently, it is not possible due to the limited deployment of this technology.
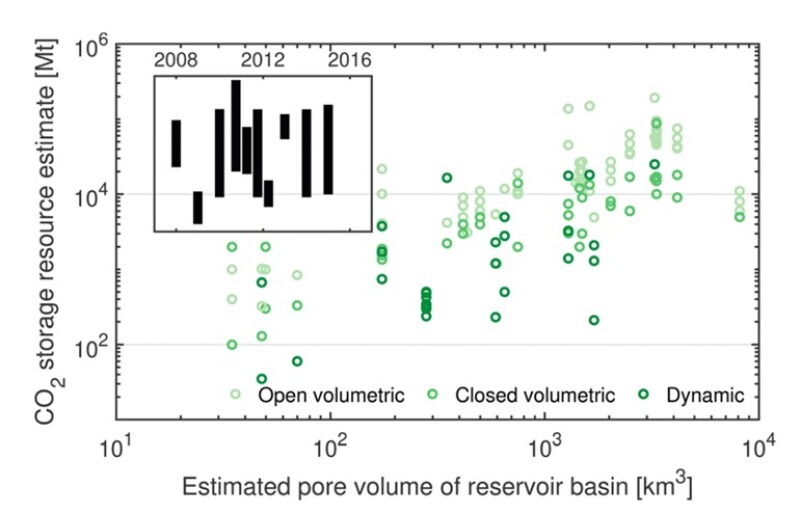
Storage resource availability does not limit upscale of CCS globally or in Europe. Analysis of the global CO2 storage supply and demand suggests that the most ambitious storage target of 1218 Gt by 2100 along a permissible growth trajectory requires a maximum storage resource base of just 2700 Gt. This is more than an order of magnitude below the 30,000 Gt of global storage resource estimates. This is within the uncertainty bounds but also indicates that the focus should be on developing a much small value of storage resources.
In Europe, the assessment using the logistic model has identified for the first time the regional geography of storage supply and demand, e.g., quantifying the potential for the North Sea to serve as a regional hub for European carbon storage demand. The modelling framework illustrates those regional plans of CO2 storage will not be limited by the availability of subsurface storage space. We always knew from previous analysis that there are plenty of storage resources in Europe, but we have now identified that the combined CO2 storage demand for Europe, for example, can be met by the subsurface reservoirs of the UK or Norway alone.
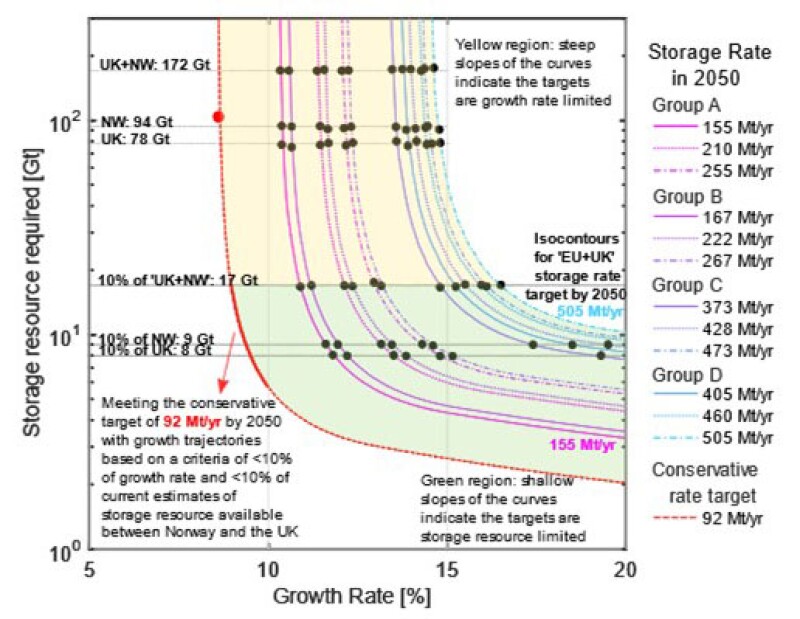
Key challenge—historically high growth rates are required to meet European targets. The key challenge revealed for Europe in the upscaling of CCS technology is that annual growth in subsurface storage rates implied by European plans is very high, often requiring over 10% annual rates of growth sustained for multiple decades to achieve 2050 storage targets. Indeed, oil production from 1901 sustained a 15% average annual growth for 40 years. However, market conditions driving the expansion of the demand for oil, which include the first World War, and few limitations ensuring safety or environmental standards, reveal the magnitude of incentivization required to achieve such growth.
The need for speed. More generally, it has been demonstrated that it is important to use logistic models to define growth trajectories that are based on both our existing knowledge of the subsurface and experience of the use of subsurface resources which ensure the outputs from integrated assessment models are grounded in realism and confidence. The combined storage resources from the UK and Norway do not significantly impact the growth rate requirements for scaleup trajectories (i.e. growths are primarily driven by the 2050 rate targets and are not limited by the availability of storage resources). Therefore, we need to deploy as fast as we can for CCS to play a role in climate-change mitigation.
References
Zahasky, C. and Krevor, S. (2020) Global Geologic Carbon Storage Requirements of Climate Change Mitigation Scenarios. Energy & Environmental Science. https://doi.org/10.1039/D0EE00674B
Zhang, Y., Jackson, C. A., Krevor, S., Zahasky, C., & Nadhira, A. A. (2022). European carbon storage resource requirements of climate change mitigation targets. International Journal of Greenhouse Gas Control 114, 103586. https://doi.org/10.1016/j.ijggc.2021.103568
Bibliography
Bachu, S., Bonijoly, D., Bradshaw, J., Burruss, R., Holloway, S., Christensen, N. P., and Mathiassen, O. M. (2007). CO2 Storage Capacity Estimation: Methodology and Gaps. International Journal of Greenhouse Gas Control, 1(4), 430–443.
Bachu, S. (2015). Review of CO2 Storage Efficiency in Deep Saline Aquifers. International Journal of Greenhouse Gas Control, 40, 188–202.
Bradshaw, J., & Dance, T. (2005). Mapping Geological Storage Prospectivity of CO2 for the World's Sedimentary Basins and Regional Source to Sink Matching. In Greenhouse Gas Control Technologies 7 (pp. 583–591). Elsevier Science Ltd.
Blondes, M. S., Brennan, S. T., Merrill, M. D., Buursink, M. L., Warwick, P. D., Cahan, S. M., and Drake II, R. M. (2013). National Assessment of Geologic Carbon Dioxide Storage Resources: Methodology Implementation (No. 2013-1055). US Geological Survey. https://pubs.usgs.gov/of/2013/1055/
Committee on Climate Change (2019). Net Zero—The UK's Contribution to Stopping Global Warming
ETI (2016). Strategic UK CCS Storage Appraisal.
European Commission (2018). A Clean Planet for All: A European Strategic Long-Term Vision For a Prosperous, Modern, Competitive, and Climate-Neutral Economy.
EU GeoCapacity (2009). Assessing European Capacity for Geological Storage of Carbon Dioxide. EU GeoCapacity Consortium.
Global CCS Institute (2018) The Global Status of CCShttps://www.globalccsinstitute.com/resources/global-status-report/.
Haszeldine, S. R. Carbon Capture and Storage: How Green Can Black Be? Science 325, 1647–1652 (2009). Hubbert, M. K. (1956, January). Nuclear Energy and the Fossil Fuel. In Drilling and Production Practice. American Petroleum Institute.
Hubbert, M. K. (1979). Hubbert Estimates from 1956 to 1974 of US Oil and Gas. In Methods and Models for Assessing Energy Resources (pp. 370–383). Pergamon.
Oil and Gas Climate Initiative (2017) Multinational CO2 Storage Resource Assessment.
IPCC (2014) Climate Change 2014 Mitigation of Climate Change. Retrieved from https://www.ipcc.ch/report/ar5/wg3/.
IPCC (2018) See chapter 2 of the special report Global Warming of 1.5 C.
Ringrose, P. S., and Meckel, T. A. (2019) Maturing Global CO2 Storage Resources on Offshore Continental Margins To Achieve 2DS Emissions Reductions. Sci Rep 9, 17944.
Rütters, H. and the CCS Europe Partners. (2013) State of Play on CO2 Geological Storage in 28 European Countries. CGS Europe Report No. D2.10. pp. 1-89.
Shell International B.V. (2018). Shell Sky Scenario.
SPE. (2017). Storage Resources Management System. Retrieved from http://www.spe.org/industry/CO2-storage-resources-management-system.php.
UK Government. (2020). Ten-Point Plan.
UNECE. (2016). Specifications for the Application of the United Nations Classification for Fossil Energy and Mineral Reserves and Resources 2009 (UNFC-2009) to Injection Projects for the Purpose of Geological Storage 1–22.
[The article was sourced from the author by TWA Editor Nihal Mounir.]