Andrei Ter-Gazarian defines energy storage as “every mechanism that enables the establishment of a reserve of energy for subsequent use independent of the initial rate of transformation.” There are many categorizations of energy storage technologies.
The attributes of good energy storage are high energy-storage capacity, high energy density, fast-charging and -discharging, high service life, low cost, high efficiency, and climate friendly.
In this article, energy storage technologies are introduced as “the talk of the town” by depicting them in the form of family trees. This article is targeted towards young energy enthusiasts looking forward to learning energy storage’s role in the carbon transition (Baxter 2006).
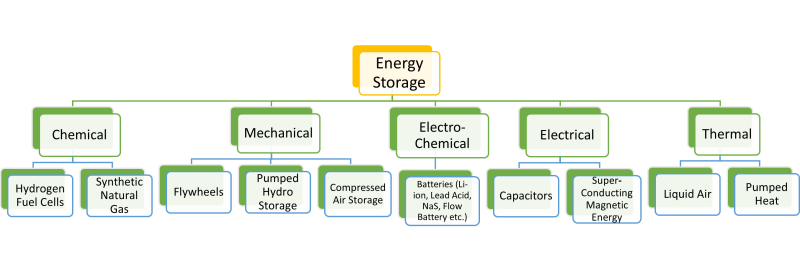
The current stores of energy are oil and gas formations. These are also forms of chemical energy storage. Energy is extracted by combustion as per requirement. The other member of chemical energy storage is hydrogen. Hydrogen acts as a fuel in reverse electrolysis and reacts with oxygen to produce energy and water. Another similar form of energy storage is batteries converting chemical energy to electrical energy in an electrochemical cell. The electrodes store energy and are also part of the reaction. Mechanical energy storage like flywheel and pumped hydro storage store kinetic and potential energies. Thermal energy storage can be sensible heat storage, thermochemical heat storage, and stored heat from phase change. Capacitors use static electric charge to store energy on the surface of electrodes, while magnetic energy is stored in superconducting coil (Demirel 2012).
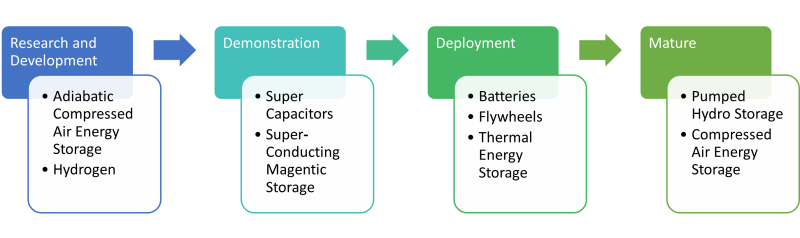
Pumped hydro storage goes back 5,000 years, and now hydro-electric turbines, which operate both as pump and generators, have efficiencies as high as 95%. Compressed air energy storage has been of significance since the inception of the gas turbine.
The current operations are adiabatic, requiring two energy inputs, one for compressing the air (which is later stored on-ground/underground) and the other for heating the cooled compressed air before combustion. The concept of adiabatic compressed air energy storage with thermal energy storage eliminates the need to heat the air pre-usage, thereby improving the efficiency of the process. Adiabatic compressed air is under research and development.
Double-layer capacitors with active material as electrodes to enhance the area for obtaining higher-capacitance and low-temperature (-100°C) superconducting coils for storing magnetic energy are in pilot stages for understanding the efficacy and gaining operational experience for commercial expansion. Other energy storage technologies like batteries and flywheels are in the deployment phase in automobile, railway, and renewable energy power backups (Sreekanth et al. 2018).
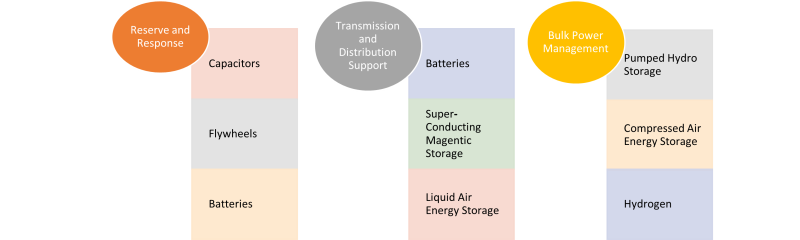
There are such varieties of energy storage as the usage differs in terms of power rating and discharge time. Capacitors and flywheels have high power density, fast response, and unlimited cycles for uninterrupted power supply. They have a power rating ranging from 10 kW to 1 MW and discharge time of seconds. Batteries are of various types, so they can provide backup reserves as well as grid support for frequency and voltage control. Batteries can function for power ratings of 10 kW to 10 MW with discharge times of seconds to hours as per battery type. For peak demand leveling, spinning reserves, renewable energy backup power, and grid-scale energy storage like pumped hydro, compressed air, and hydrogen can be used. This bulk power management has a very high power rating of 100 MW to 1 GW with a discharge time of days to months for seasonal/daily variations (Howitt 2018).
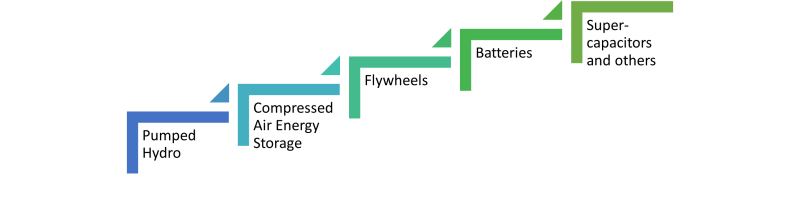
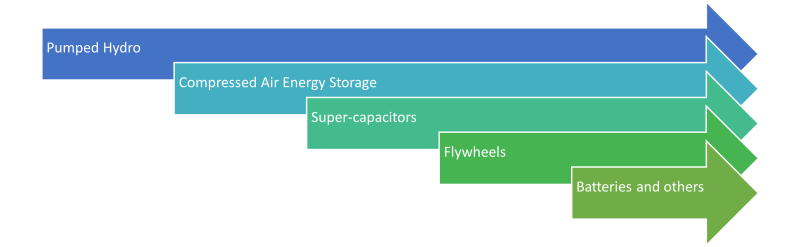
Increasing lifetime (with arrow length)
Pumped hydro storage has an elastic cost range depending on size, operating hours, materials used for construction, labor cost, etc. Although the capital costs and the pay time (20–40 years) are high, the lifetime of a pumped hydro storage is 100 years. The cost of compressed air energy storage depends on the availability of natural underground storage and size. The higher the size, the more cost-effective compressed air storage is compared to pumped hydro storage. The maximum storage period is more than 1 year, as the losses are minimal. Flywheels store high power for shorter periods. The cost of flywheels depends on the rotor design stresses for maximizing the stored energy. The cost of supercapacitors depends on the active material used to increase area and the capacitance for higher energy densities. Both flywheels and supercapacitors are used for short-term energy supply for unlimited charge discharge cycles. Batteries vary in cost and lifetime depending on the type, working process electrodes, and electrolyte. They have a shorter cycle lifetime as compared to capacitors and flywheels (Howitt 2018).
Stay tuned for Part 2 which continues the discussion advances in energy storage.
References
Energy Storage: A Nontechnical Guide (2006) by Richard Baxter
Energy: Production, Conversion, Storage, Conservation, and Coupling (2012) by Yaşar Demirel
Gigawatt Scale Storage for Gigawatt Scale Renewables (2018) by Mark Howitt
Feasibility Analysis of Energy Storage Technologies in Power Systems for Arid Region (2018) by K. J. Sreekanth, R. Al Foraih, A. Al-Mulla, and B. Abdulrahman