As the global energy sector pivots toward cleaner alternatives, underground hydrogen storage (UHS) is gaining traction as a pivotal component of the low-carbon hydrogen economy. While subsurface storage offers an attractive solution for balancing renewable energy intermittency, its successful deployment requires far more than identifying formations with sufficient pore space.
From geochemical stability to geomechanical integrity, the selection of suitable storage sites demands a holistic, multidisciplinary approach. Recent studies are expanding our understanding of the nuanced variables affecting UHS performance, revealing that factors like microbial activity, reservoir stress behavior, and even hydrogen production potential from geological formations play critical roles.
This article synthesizes current research themes that go beyond the conventional site screening criteria, offering a broader lens through which to evaluate and optimize subsurface hydrogen storage.
Building on this foundational understanding, Fig. 1 illustrates the key geological factors that influence hydrogen behavior within depleted oil and gas fields, highlighting the complexity and interdependence of subsurface processes in UHS systems.
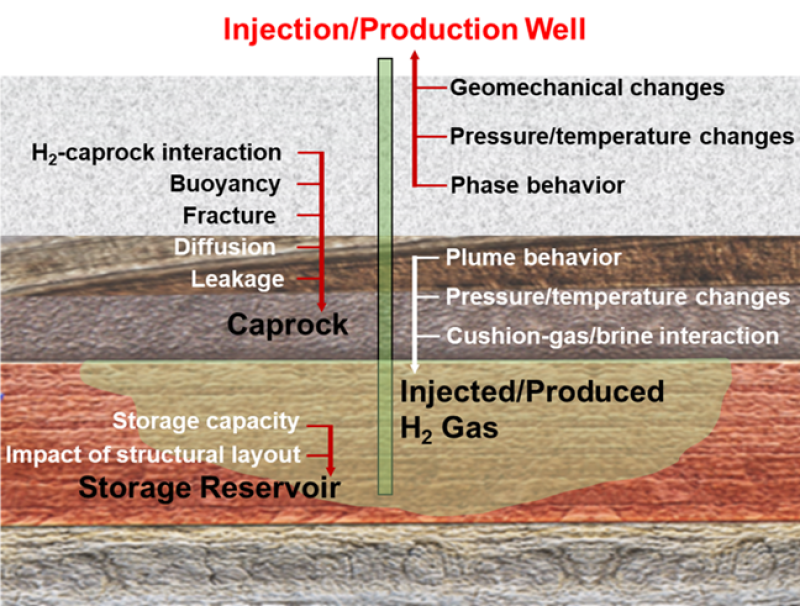
Sekar et al. (2023) highlights that while UHS is a promising solution to support the hydrogen economy, it poses significant engineering challenges that demand multidisciplinary approaches. The review assesses the suitability of depleted oil and gas fields (DOGFs) and saline aquifers for UHS, noting that these formations are still under evaluation. The review covers geochemical, microbiological, experimental, and modeling studies that influence storage capacity by affecting petrophysical and hydrodynamic properties. Geochemical interactions, such as contamination from CO2 or sulfur compounds like hydrogen sulfide from pyrites, may alter reservoir characteristics. However, much of the existing research suggests these reactions cause minimal hydrogen losses, supporting the viability of UHS from a geochemical standpoint.
Simulation-based feasibility studies generally show positive results for UHS in DOGFs, with key considerations including reservoir structure, sealing mechanisms, cushion gas selection, and in-situ pressure. Despite progress in geochemical and reservoir modeling, there remains a significant gap in understanding the geomechanical behavior of formations under hydrogen storage conditions. This is critical due to hydrogen’s high diffusivity and leakage risk. Factors such as rock failure criteria, including changes in friction angle and cohesion in the presence of hydrogen, need closer examination. Bibliometric analysis reveals that UHS is still a developing field with dynamic research themes, underscoring the need for continued and integrated scientific efforts.
Building on these knowledge gaps, recent studies have begun to explore the geomechanical implications of UHS more closely. Preliminary work on geomechanical modeling by Okoroafor et al. (2024) suggested that hydrogen storage in saline aquifers has a larger probability of induced seismicity (assuming the rocks are critically stressed) and uplift than in depleted gas reservoirs. When comparing hydrogen storage in depleted gas fields to natural gas storage in depleted gas fields, the former results in larger stress changes in the reservoir and on the caprock. In the presence of a leakage pathway, hydrogen, being more mobile, moves faster than methane and, thus, during injection, has less gas in place. This remains the case until the leakage path is filled with gas and there is no further outlet for the fluids.
Following geomechanical analysis, it is equally important to evaluate storage performance in terms of hydrogen losses, as these directly impact recovery efficiency and long-term viability of storage operations. Work by Indro et al. (2024) compiles hydrogen loss data from various sources and models recovery factors and productivity indices across three types of water-bearing geological formations: shallow reservoirs with normal and high geothermal gradients, and deep saline aquifers. Simulations were conducted for 30-, 90-, and 180-day storage cycles, incorporating estimated losses from literature. Results indicate hydrogen recovery factors ranging from 13 to 83%, depending on reservoir type, cycle duration, and depth.
Deep saline aquifers consistently offer higher recovery across all cycles but initially lower productivity indices, which improve for long-term storage (more than150 days). Reservoirs with high geothermal gradients and temperatures above 120°C are viable for hydrogen storage if adequate cushion gas is used. Overall, depth, temperature, and salinity were more influential on hydrogen losses than mineralogy.
Building on the broader understanding of hydrogen losses, site-specific evaluations are essential to identify optimal storage conditions and maximize withdrawal efficiency in real-world settings. Sekar et al. (2024a) aimed to evaluate the suitability of saline reservoirs for UHS storage with a focus on maximizing withdrawal efficiency, using California's Sacramento Basin as a case study. Through extensive numerical simulations, key favorable reservoir characteristics were identified, including steep dips (up to 15°), low pressures, high porosity (more than 20%), and high permeability. A comprehensive site screening and ranking framework was developed and applied to five formations within the basin, demonstrating its effectiveness in identifying optimal storage sites. Unlike previous frameworks focused on CO₂ or depleted gas fields, this study emphasized both storage and efficient retrieval of hydrogen, making the methodology broadly applicable to other basins.
Collectively, these studies underscore the importance of a holistic evaluation approach that incorporates geochemical stability, geomechanical safety, and hydrogen loss mitigation to ensure an effective UHS. Building upon these insights, Sekar et al. (2024b) presents the development of a comprehensive, multistage screening and site ranking framework for hydrogen storage in saline aquifers. Using numerical reservoir simulations, the study modeled injection, storage, and withdrawal scenarios, and conducted a sensitivity analysis to identify key variables influencing hydrogen storage performance. Initial disqualifying criteria were used to eliminate unsuitable sites, followed by a first stage ranking based on productivity and storage integrity. The second and third stages incorporated literature-based data on geochemical and microbial reactions, as well as transport-related hydrogen losses. Applied to saline aquifers in the Sacramento Basin, the framework revealed that different criteria alone may suggest different optimal sites. However, integrating weighted factors enabled the identification of the most favorable site—balancing maximum recovery and productivity with minimal losses and integrity risks.
Building directly on the multistage screening framework, Sekar and Okoroafor (2024) further translates the methodology into a practical, open-source tool—Hydrogen Underground Storage in Porous Media (HUST)—designed to streamline site selection by integrating geochemical, microbial, and hydrodynamic considerations into a unified decision-making platform with a case application in the Sacramento Basin. Unlike previous approaches, this tool integrates geochemical and microbial interactions alongside hydrodynamic and operational factors through a three-stage screening process. Stage 1 focuses on productivity index and key variables affecting hydrogen recovery; Stage 2 accounts for hydrogen losses from geochemical reactions; and Stage 3 addresses microbial-induced losses.
The tool allows for weighted evaluation of each factor, ensuring a more robust site selection. Results show that relying solely on hydrodynamic criteria may lead to poor site choices, as high-permeability sites may still suffer significant hydrogen losses. By incorporating all relevant processes, the tool identifies sites that balance storage capacity, productivity, and minimal hydrogen loss, filling a critical gap in UHS-porous media site selection methodologies.
Following the technical screening and identification of optimal sites using the HUST tool, the focus shifts toward evaluating the economic viability of UHS—assessing the cost-effectiveness of storing hydrogen from various production pathways to support informed, site-specific deployment decisions. Tayyib et al. (2024) highlights UHS as an economically viable solution for mitigating renewable energy curtailment. Using Electric Reliability Council of Texas (ERCOT) curtailment data and reservoir simulations, UHS was compared with synthetic geothermal heat storage in terms of energy recovery, cost, and emissions. UHS demonstrated higher energy recovery (29–57%) despite a slightly higher cost ($102–115/MWh), while geothermal storage, though cheaper ($19–73/MWh), recovered significantly less energy (6–17%). These results position UHS as a more effective option for large-scale, long-duration energy storage to enhance grid reliability and reduce renewable energy waste.
While UHS is crucial for stabilizing renewable energy integration and ensuring long-term energy reliability, securing a sustainable, low-carbon hydrogen supply is equally vital. The elevated levelized cost of hydrogen (LCOH) observed in the previous study largely stems from the high cost of hydrogen production. To complement storage efforts, it is therefore essential to explore innovative and sustainable hydrogen generation pathways. One promising avenue is leveraging natural geological processes like the serpentinization of ultramafic rocks, which enables low-carbon, in-situ hydrogen production directly from the subsurface. This emerging method offers a decentralized, scalable solution that can synergize with storage strategies, strengthening the overall hydrogen economy.
Building on the need for sustainable hydrogen supply pathways, a study by Sekar and Okoroafor (2025) shifts focus from storage to generation—demonstrating how engineered subsurface systems, particularly those leveraging ultramafic rock formations, can serve as viable sources of low-carbon hydrogen through geochemical activation and targeted reservoir stimulation. This study presents a preliminary model for hydrogen generation from hydraulically stimulated ultramafic rocks using a coupled thermohydrochemical framework that simulates heat transfer, fluid flow, and geochemical reactions during induced serpentinization. Simulations compared hydrogen yields from stimulated vs. unstimulated rocks, revealing a twofold increase in production with stimulation, especially in vertical well configurations.
While longer horizontal wells initially enhanced gas output, they also promoted water production, leading to diminishing gas yields over time. Catalysis significantly improved hydrogen generation by accelerating reaction rates and reducing activation energy. With a seal/caprock in place, the ultramafic rock layer acted as a temporary hydrogen reservoir. Sensitivity analysis showed fault thickness had minimal effect in vertical wells but significantly impacted production in horizontal wells—enhancing yield in adjacent porous layers. The findings suggest that optimized configurations—including horizontal wells in ultramafic layers, catalytic enhancement, thick faults, and nearby porous zones—can maximize hydrogen generation, migration, and accumulation. The study highlights the need for further data collection, model refinement, and advanced simulation tools, marking a significant step toward engineering efficient ultramafic hydrogen generation systems.
UHS stands at the forefront of enabling a resilient, low-carbon hydrogen economy, but its effective deployment hinges on far more than pore space availability. This article underscores the critical importance of a multidisciplinary lens—one that integrates geochemical stability, geomechanical integrity, microbial interactions, and economic viability—to holistically evaluate and optimize UHS systems.
From simulation-based site screening and geomechanical risk assessment to advanced modeling of hydrogen losses and recovery efficiency, recent research has illuminated both the opportunities and challenges of subsurface hydrogen storage. Moreover, as storage alone cannot sustain the hydrogen value chain, innovative generation methods—such as engineered serpentinization of ultramafic rocks—present scalable, low-emission solutions to complement UHS efforts. Together, these advances signal a paradigm shift: toward integrated hydrogen infrastructure that leverages both subsurface storage and production to stabilize renewables, reduce emissions, and realize the full potential of a global hydrogen economy.
For Further Reading
Review of Reservoir Challenges Associated With Subsurface Hydrogen Storage and Recovery in Depleted Oil and Gas Reservoirs by L. Sekar, R. Kiran; Indian School of Mines; R. Okoroafor, Texas A&M University; A. Wood, DWA Energy Ltd.
SPE 220971 Evaluating Carbonate Reservoir Rocks for Underground Hydrogen Storage: A Comprehensive Laboratory Approach by H. Galvis-Silva, R. Okoroafor, L. Sekar, Texas A&M University.
Underground Hydrogen Storage in Porous Media: The Role of Petrophysics by R. Okoroafor, H. Galvis, Texas A&M University
A Compilation of Losses Related to Hydrogen Storage in Porous Media: Implications for Hydrogen Recovery and Productivity From Saline Aquifers by A. Indro, Los Alamos National Laboratory; L. Sekar, C. Ikeokwu, R. Okoroafor, Texas A&M University, G. Matey-Korley.
Prospects for Underground Hydrogen Storage in Saline Reservoirs: A Case Study of Sacramento Basin by L. Sekar, H. Galvis, and R. Okoroafor, Texas A&M University.
Development and Implementation of a Comprehensive Multistage Ranking Criteria for Underground Hydrogen Storage in Saline Aquifers by L. Sekar, H. Silva, and R. Okoroafor, Texas A&M University.
SPE 220977 Development and Application of HUST: A Hydrogen Underground Storage Screening and Ranking Tool Incorporating Operational Considerations, Geochemical and Microbial Reactions by L. Sekar and R. Okoroafor, Texas A&M University.
Integrated Techno-Economic and Life-Cycle Assessment of Subsurface Energy-Storage Technologies for Renewable Energy by D. Tayyib, L. Sekar and R. Okoroafor, Texas A&M University.
SPE 223864 Thermohydrochemical Modeling of Hydrogen Generation from Stimulated Ultramafic Rocks by L. Sekar, R. Okoroafor, Texas A&M University.