The oil and gas industry is often criticized for its slow pace to develop and implement technology. Nevertheless, the vision and drive of the scientists and engineers in our industry who, since the 1970s, have devoted entire careers to conceiving and developing one of the most important enhanced-recovery methods for unconventional heavy oil/bitumen can only be admired. Steam-assisted gravity drainage (SAGD) is a prime example of how structured research and development (R&D) has led to the commercial implementation of technology, helping unlock hydrocarbon resources to fulfill society’s energy demands.
Producing more, faster or, simply, producing what was previously thought impossible are the driving forces behind most R&D initiatives in our industry. The province of Alberta, Canada, holds approximately 170 billion bbl of recoverable bitumen reserves. This resource base makes Canada’s petroleum reserves the second largest in the world. However, the majority of this bitumen is trapped at very shallow depths and immobile at undisturbed reservoir conditions because of its extremely high viscosities. These conditions led the industry to conclude that most of these resources were impossible to produce.
In fact, approximately 20% of these reserves are shallow enough to be produced by surface mining. However, to produce bitumen from depths beyond those accessible by surface-mining methods requires a different approach: in-situ recovery. The high undisturbed bitumen viscosities (more than 50,000 cP at room temperature) require the addition of heat to mobilize the fluid. However, the shallow depths [70 to 600 m total vertical depth (TVD)] impose the challenge of adding this energy without any uncontrolled breakthrough to the surface. These were the main driving forces behind the development of an in-situ-recovery method that could convey sufficient energy to mobilize the bitumen and achieve economic recovery factors, while being gentle enough (having sufficiently low pressure) to allow application in shallow reservoirs. SAGD fills these requirements. Its effectiveness has been proven through laboratory, analytical, numerical, and field applications.
A Bit of History
Inventions and scientific discoveries, especially the most important ones, have frequently brought out controversy over who led the way. The credit for invention of the SAGD bitumen-extraction process has been no different (Edmunds and Chhina 2001). However, the oil industry recognizes Roger Butler, from Imperial Oil, as the inventor of this method (Butler 1980). He was the first to articulate the idea of integrating the SAGD concept with horizontal-well technology—then in its infancy—to achieve economic feasibility.
In 1978, at Cold Lake, Alberta, Canada, Imperial Oil drilled the first modern horizontal producing well paired with a vertical steam-injection well. In a later interview (Lowey 2006), Butler described the success, “The oil came at about the right rate—I felt pretty darn good!” From that initial success, the SAGD concept was formally developed along with process mechanisms and mathematical modeling to forecast energy consumption and hydrocarbon production.
The Alberta Oil Sands Technology Research Authority (AOSTRA) carried out proof-of-concept field tests with SAGD in the 1980s, with the first-ever (paired) twin SAGD wells drilled in 1987 at AOSTRA’s Dover Underground Test Facility (UTF). The UTF consists of a series of underground mine tunnels drilled at 140 m TVD, in limestone below the target reservoir, from which three pairs of 600-m horizontal wells were drilled (Komery et al. 1995). At that time, the technology to drill well pairs from surface, using conventional drilling-rig technology, did not exist. The production success and field experience obtained from this project was essential for understanding SAGD field performance and the challenges that would need to be overcome for commercial application.
Most of the commercial SAGD projects in Canada have come on stream since 2000. Currently there are about a dozen projects in operation, with total oil production of approximately 240,000 B/D. The industry forecast indicates that output from SAGD projects could triple over the next decade.
The Process
The SAGD process consists of a horizontal well, or a series of vertical wells, injecting steam above another horizontal well that acts as a producer. Fig. 1 details the main process mechanisms. As steam is injected, it forms a steam-saturated chamber. At the edges of the chamber, the steam condenses, liberating its latent heat and, thus, mobilizing the bitumen by reducing its viscosity. Then gravity takes charge, driving the condensed water and hydrocarbons to the production well at the bottom of the chamber (Butler 1994).
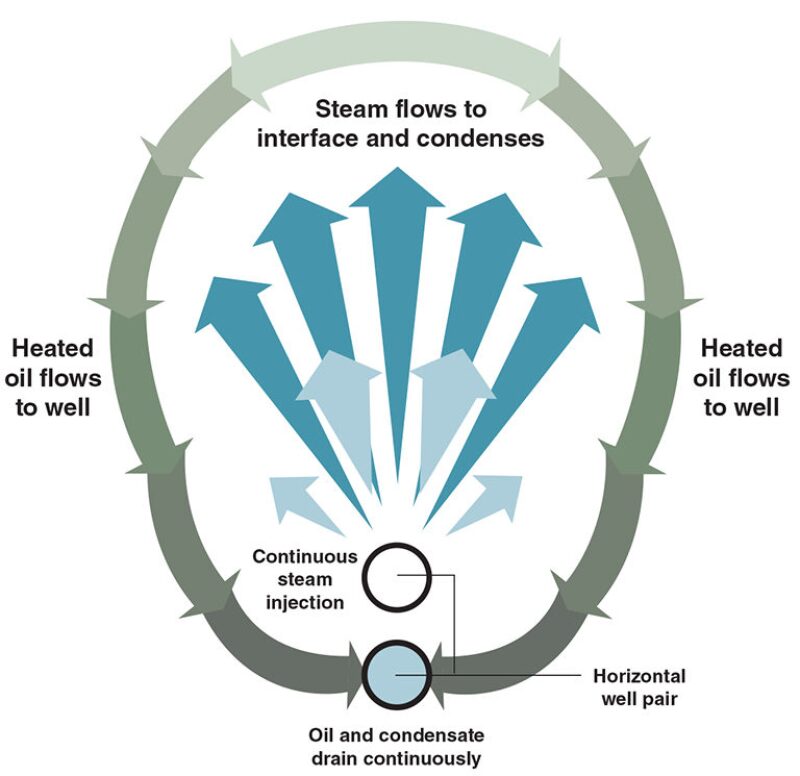
The expansion of the steam chamber, in the longitudinal and axial directions relative to the wellbore, can be rather irregular but quite rapid, until it finds the top of the reservoir. The process relies on the heated bitumen being produced as the steam chamber grows. Uniform development of the steam chamber remains one of the most critical challenges of the SAGD process because of its direct impact on bitumen recovery.
The SAGD process takes place in three distinct phases: startup, or circulation; normal SAGD operation; and wind down. The startup is aimed at mobilizing the bitumen close to and between the injector and producer to establish “communication” between the wells. The most widely used method for startup is circulating steam in both wells for as long as 90 days. Normal operation involves injecting steam and producing bitumen to form the steam chamber above the well pairs. This provides access to the maximum amount of resources within the drainage area. This phase lasts as many years as necessary, so that the maximum amount of oil is recovered from the drainage volume. Finally, the wind down consists of a series of operations aimed at reducing the amount of steam injected and using auxiliary operating patterns to maximize recovery.
Geology and Reservoir
Most SAGD applications are on shallow, unconsolidated sandstone reservoirs with high permeability. However, recently a significant quantity of heavy oil has been identified in Alberta’s carbonate Grossmont formation, which some believe can be economically exploited with SAGD.
Reservoir screening criteria have been the subject of some debate in the industry (Albahlani and Babadagli 2008). However, there is a general agreement that resource quality remains the most critical factor in SAGD project performance. Net-pay thickness, oil saturation, and porosity are the main properties required to estimate the reserve base of a project and forecast recovery factors. Vertical permeability can also influence the effectiveness of the recovery process. For example, the presence of shale layers, or breccia, within the sandstone formation can hamper the rise of the steam chamber and/or the producer/injector communication, if located between the injector and producer wells.
The overburden, or cap rock, has special relevance for most SAGD projects. It must provide a barrier to prevent the loss of steam to shallower strata or, in the worst case, to surface. The geomechanical competence of the cap rock must be carefully assessed. If the overburden allows for leakage of steam to surface, it will be a catastrophic accident resulting in serious impact to the environment and safety. If the steam leaks to shallower strata, it would seriously affect the expansion of the chamber and, thus, thermal efficiency and ultimate recovery.
The presence of top or bottom water and/or gas caps must be considered in the SAGD development plan. Ongoing research is improving our understanding of how these fluid interfaces affect thermal efficiency and bitumen recovery (Edmunds and Chhina 2001 and Peterson et al. 2009).
Well Construction
Directional-drilling technology evolution has been a key enabling factor in the commercial implementation of SAGD. Two parallel horizontal wells are drilled to vertical depths between 90 and 600 m, with 4 to 7 m of vertical offset and up to 1000 m of horizontal displacement. Due to their shallow vertical depths, some of these wells require slant drilling from the surface. Typically, the production well is drilled first and placed as close as possible to the bottom of the reservoir.
In 1993, the technology to drill parallel horizontal wells was developed. The first SAGD well pair was drilled using magnetic-ranging/-guidance technology, which refers to the measurement of the relative position of one well with respect to another. It determines the distance and orientation from the well being drilled (injector) to the reference well (producer). The determination is based on measuring the magnetic signature from the target/reference well, which may be induced and measured by several methods (Grills 2002).
SAGD wells must be designed to withstand the harsh environment of this process. Integrity and reliability must be balanced with the requirement to minimize capital expenditure. Typical SAGD well designs are shown in Fig. 2. The intermediate casing is the main barrier for isolating the bottomhole SAGD environment from the surface, while the integrity of the production liner is crucial to avoid sand production. Therefore, the selection of adequate cement for thermal conditions and proper thermal casing design (steel and connection selection) are critical (IRP 3, Enform 2002; Kaiser 2009; and Nowinka and Dall’Acqua 2009).
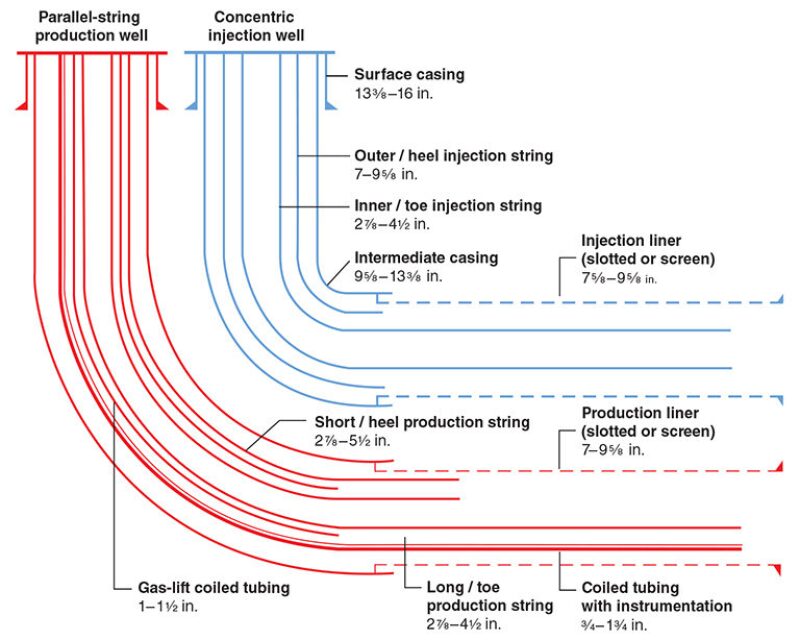
Well-completion designs can vary between operators and even within projects. The industry is still in an evaluation phase, and different completion configurations are being implemented. However, the general trend is to allow for steam injection or bitumen production at two or more points along the horizontal wellbore. As illustrated in Fig. 2, the typical completion designs are dual parallel, or concentric, strings with tubing and annulus flow paths.
The unconsolidated nature of the sandstones in which most SAGD projects are carried out has led to the requirement of sand control in both wells. Slotted liners are the most widely used sand-control method, with stand-alone screens a distant second. This preference probably reflects the lower cost of slotted liners, along with research efforts (Bennion et al. 2009; and Kaiser et al. 2002) that have improved their design and performance. However, as more challenging reservoirs are targeted and more operational issues arise, the industry continues to seek other competitive sand-control options.
Production Operations and Control
SAGD operations require monitoring strategies aimed at controlling the downhole process, to avoid operational issues and maximize efficiency and recovery. Producer wells are fitted with multiple temperature-measuring devices along the horizontal wellbore. Thermocouples are the preferred choice because of their reliability and lower cost; however, fiber-optic technology has been field tested widely and implemented as well. Pressure monitoring is also employed in producer wells through bubble tubes, open-annulus gauges, or, more recently, fiber-optic gauges. Typically, the injector wells have less instrumentation.
The main objective of temperature monitoring in the producer well is steam-trap control. The goal is to maintain the steam/liquid interface between the wells. This is achieved by managing the difference between the producer bottomhole temperature and the saturated-steam temperature at the injector bottomhole pressure (subcool) to maintain a balance that keeps the interface between the wells. Optimum SAGD requires the steam chamber to be drained so that liquid does not accumulate over the producer—reducing production rates—but avoiding steam production because it jeopardizes the integrity of the producer well (Edmunds 1998).
Monitoring steam-chamber development is the single most important task for understanding the SAGD process and diagnosing recovery efficiency. Along with real-time in-well monitoring, the following methods are combined to estimate steam-chamber growth:
- Fully instrumented vertical observation wells along the horizontals at each pad
- Surface-deformation measurement technologies, such as heave monuments, tiltmeters, and interferometric-synthetic-aperture radar
- Seismic methods, such as 4D and microseismic mapping
- Production history matching
The steam-chamber operating pressure can also be controlled to improve efficiency and recovery. However, this will also affect the selection of the produced-fluids’ lift method. During the initial SAGD operation phase, most projects operate at pressures high enough to use steam or gas lift (Kisman 2001). The use of gas lift for SAGD has features that differ from its conventional applications and requires proper assessment to assure success (Handfield et al. 2008). As the SAGD project matures, the tendency is to operate at lower chamber pressure, which requires different artificial-lift methods. In recent years, R&D efforts in high-temperature mechanical-lift equipment have resulted in the ability to pump high volumes of fluids at elevated temperatures (220–260°C) with tolerance to steam, gas, and sand/fines production. Most installations have involved “state-of-the-art” electrical submersible pumps and metal progressive-capacity pumps.
Performance and Challenges
Most companies have their own evaluation methods for SAGD performance. However, steam-to-oil ratio (SOR) is commonly used as the key performance indicator to benchmark project efficiency. SOR indicates the volume of steam required to produce a certain amount of oil. Although it does not truly reflect energy-use efficiency, it remains a widely used industry metric. The aim is to minimize SOR, where values in the 2.0 to 3.5 range are considered good performance. Currently, the best performing SAGD projects by this criterion are: Devon’s Jackfish, Cenovus’ Foster Creek and Christina Lake, and Suncor’s Firebag projects.
Other performance indicators are bitumen production rates and recovery factors. The industry average bitumen production per well pair is between 400 and 1,000 B/D with ultimate recovery factors higher than 50% (Handfield et al. 2008).
The current trend for most commercial projects is to increase the thermal efficiency and bitumen recovery of the process through enhanced techniques or SAGD variations, such as nonparallel-well geometrical configurations, additional wells, solvent injection, steam-distribution optimization, and inflow control.
Despite the successful commercial implementation of SAGD technology, the industry still has two major challenges to overcome: dependence on natural gas and environmental impact. Most SAGD projects still rely on natural gas as the energy source for producing steam. The volatility of natural-gas prices, especially in North America, and the long-term supply uncertainty directly impact the economic performance and overall feasibility of SAGD projects.
Finally, SAGD’s carbon footprint and water requirements are quite substantial, mainly due to the steam-generation process. Cogeneration, brackish-water use, water recycling, and other enhancements are being implemented or considered to minimize the environmental impact of SAGD. However, additional efforts are required because more stringent regulatory requirements are likely forthcoming. Furthermore, SAGD’s negative perception by a portion of the general public remains an important driving force to clean up the image of SAGD and guarantee the sustainable development of the technology. TWA
References
Albahlani, A.M. and Babadagli, T. 2008. A Critical Review of the Status of SAGD: Where Are we and What Is Next? Paper SPE 113283 presented at the SPE Western Regional and Pacific Section AAPG Joint Meeting, Bakersfield, California, 29 March–2 April.
Bennion, B., Gupta, S., Gittins, S., and Hollies, D. 2009. Protocols for Slotted Liner Design for Optimum SAGD Operation. JCPT 48 (11): 21–26.
Butler, R. 1980. Method for Continuously Producing Viscous Hydrocarbons by Gravity Drainage While Injecting Heated Fluids. US Patent No. 4,344,485.
Butler, R. 1994. Steam-Assisted Gravity Drainage: Concept, Development, Performance and Future. JCPT 33 (2): 44–50.
Edmunds, N. 1998. Investigation of SAGD Steam Trap Control in Two and Three Dimensions. Paper SPE 50413 presented at the SPE International Conference on Horizontal Well Technology, Calgary, 1–4 November.
Edmunds, N. and Chhina, H. 2001. Economic Optimum Operating Pressure for SAGD Projects in Alberta. JCPT 40 (12): 13–17.
IRP 3, Heavy Oil and Oil Sands Operations, 2002, Calgary: Enform.
Handfield, T., Nations, T., and Noonan, S.G. 2008. SAGD Gas Lift Completions and Optimization: A Field Case Study at Surmont. Paper SPE 117489 presented at the International Thermal Operations and Heavy Oil Symposium, Calgary, 20–23 October.
Grills, T. 2002. Magnetic Ranging Technologies for Drilling Steam Assisted Gravity Drainage Well Pairs and Unique Well Geometries—A Comparison of Technologies. Paper SPE 79005 presented at the SPE International Thermal Operations and Heavy Oil Symposium and International Horizontal Well Technology Conference, Calgary, 4–7 November.
Kaiser, T. 2009. Post-Yield Material Characterization for Thermal Well Design. SPEJ 14 (1): 128–134. SPE 97730.
Kaiser, T.M.V., Wilson, S., and Venning, L.A. 2002. Inflow Analysis and Optimization of Slotted Liners. SPE Drill. & Compl. 17 (4): 200–209. SPE 80145.
Kisman, K.E. 2001. Artificial Lift: A Major Unresolved Issue for SAGD. JCPT 42 (8): 39–45.
Komery, D.P., Luhning, R.W., and O’Rourke, J.C. 1995. Towards Commercialization of UTF Project Using Surface Drilled Horizontal Wells. Paper 95-60 presented at the 46th Annual Technical Meeting of the Petroleum Society of the Canadian Institute of Mining, Banff, Alberta, Canada, 14–17 May.
Lowey, M. 2006. An Interview With Roger Butler, Alberta Oil 2 (2), http://www.albertaoilmagazine.com/2006/04/an-interview-with-roger-butler/, last accessed 12 May 2010.
Nowinka, J. and Dall’Acqua. D. 2009. New Standard for Evaluating Casing Connections for Thermal Well Applications. Paper SPE 119468 presented at the SPE/IADC Drilling Conference and Exhibition, Amsterdam, 17–19 March.
Peterson, J.A., Riva, D.T., Connelly, M.E., Solanki, S.C., and Edmunds, N.R. 2009. Conducting SAGD in Shoreface Oil Sands With Associated Basal Water. Paper CIPC 2009-128 presented at the Canadian International Petroleum Conference, Calgary, 16–18 June.