CO2 Enhanced Oil Recovery: Background
With 50% to 65% of the original oil in place (OOIP) remaining in many reservoirs after primary and secondary recovery, more operators and governments are considering the use of enhanced oil recovery (EOR) processes as an opportunity whose time has come. Additionally, in the face of global warming, the prospect of CO2 sequestration has arisen with the potential of sequestering large quantities of CO2 in depleted oil fields as a means of preventing its release into the atmosphere.
The concept of injecting gas into a formation to stimulate recovery of residual oil is not new. Successful laboratory gas-injection experiments generated a lot of optimism in the 1950s, but by the 1970s field experiments yielded only moderate recoveries of 5% to 10% of the remaining OOIP. Viscous fingering, solvent channeling, and reservoir heterogeneity were found to be the main culprits for the disappointing field performance. Early efforts were directed at developing methods to “improve” the injected gas’s mobility and volumetric sweep efficiency, and included the water-alternating-gas (WAG) process, using foaming agents and polymers. Much of these research efforts continue today in universities and other research laboratories. One concern, however, is that the research favors modeling work rather than experimental development of new concepts and processes.
In a recent study for the US Department of Energy (DOE), Advanced Resources International identified four advanced CO2 EOR technologies:
- Higher pore volume of CO2 injection
- Vertical gravity stable gas injection with horizontal wells for production
- Miscibility development
- Effective mobility control in horizontal floods
This article discusses the gas-assisted gravity drainage (GAGD) technology being developed at Louisiana State University with the support of a DOE research grant.
Gas-Assisted Gravity Drainage
The GAGD process is shown schematically in Fig. 1. CO2 injected in vertical wells accumulates at the top of the pay zone and displaces oil, which drains to a horizontal producer straddling several injection wells. As injection continues, the CO2 chamber grows downward and sideways, resulting in larger portions of the reservoir being swept without any increase in water saturation in the reservoir. This maximizes the volumetric sweep efficiency. The gravity segregation of CO2 also helps in delaying, or even eliminating, CO2 breakthrough to the producer as well as preventing the gas phase from competing for flow with oil.
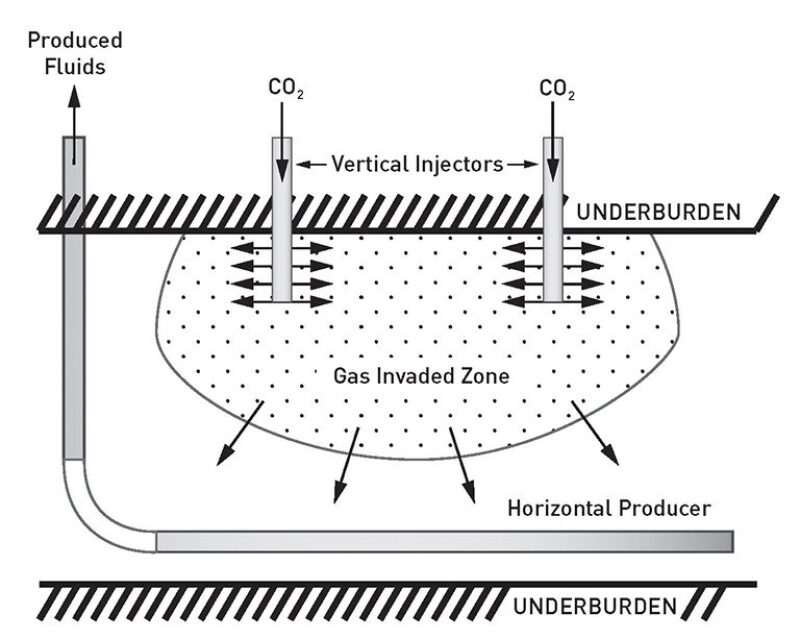
Additionally, keeping the pressure above the minimum miscibility pressure (MMP) maximizes the recovery efficiency. This keeps the interfacial tension between the oil and the injected CO2 low, which in turn results in large capillary numbers and low residual oil saturations in the swept region. If the formation is water-wet, water is likely to be held back in the rock pores by capillary pressure while oil will be preferentially displaced by CO2. If the formation is oil-wet, continuous films of oil will help create drainage paths for the oil to flow to the horizontal producer.
The GAGD process has the additional advantage of increased oil saturation and consequently improved oil relative permeability near the producing wellbore.
The process makes use of existing vertical wells in the field for CO2 injection and calls for drilling a long horizontal well for producing the draining oil. The drilling costs of horizontal wells have been significantly reduced in recent years due to technology advances. Our ongoing experimental studies of GAGD have shown recoveries in the range of 73% to 85% remaining OOIP in its secondary immiscible mode and near complete recoveries in miscible modes. The mechanisms of displacement, gravity drainage, and film flow combine to yield such high recoveries even in immiscible gas injection experiments. This opens up a vast arena for further research and practical applications on the use of flue gases for immiscible GAGD EOR applications without having to separate the CO2, which constitutes about 75% of the cost. The CO2 GAGD process is being piloted in a Louisiana field and the results are awaited.
CO2 Sequestration
Another research area often affiliated with CO2 EOR is carbon capture and storage (CCS). The idea behind CCS is that human-generated (anthropogenic) greenhouse gases are separated and captured from concentrated sources such as power plants, refineries, chemical plants, or cement plants. These gases are then injected into the subsurface in active or abandoned oil and gas reservoirs, deep underground coal seams, certain volcanic formations, or deep saline aquifers.
The cost of separating and capturing the greenhouse gases as well as of the assessment and assignment of liability for the long-term integrity of sequestration sites are the primary factors limiting widespread implementation of CCS. However, there are active areas of research associated with technologies more aligned with oil- and gas-related topics. Chief among these technologies are monitoring the fate of injected CO2, evaluating caprock and wellbore integrity, understanding sequestration storage mechanisms, and characterizing storage formation parameters with limited data.
The cost of separating and capturing the greenhouse gases as well as of the assessment and assignment of liability for the long-term integrity of sequestration sites are the primary factors limiting widespread implementation of CCS.
The primary difference between using CO2 for enhanced oil recovery and for sequestration is that while EOR processes seek to utilize the CO2 efficiently, there is no requirement to ensure that the CO2 remains permanently underground. Ensuring wellbore integrity at the sequestration site relies on understanding and extending oilfield technologies of well construction, cement chemistry, and cement evaluation. Monitoring the fate of the injected CO2 also relies on oil- and gas-related geophysical measurements. For sequestration, the site may undergo depletion followed by repressurization. Since the caprock ensures the storage capability of the site, whether the depletion or pressurization of the formation breaches the integrity of the caprock needs to be evaluated. Modeling of the sequestration storage mechanisms of adsorption, solubility trapping, capillary trapping, and mineralization requires both adequate models for each mechanism and laboratory measurements to calibrate the models. The dominant mechanism for storage depends on pore structure, mineralogy, and rock and fluid properties. Formation characterization for sequestration differs only slightly from oilfield exploration well characterization in that formation mineralogy must be modeled in addition to the more traditional rock and fluid properties.
In the US, funding for CO2 sequestration research is primarily through the Regional Partnership program at the DOE. The seven Regional Partnerships have field projects designed to demonstrate full-scale implementation of CCS in each of the formation types. Budgets for these projects are dominated by the cost of implementation and monitoring. Additional funding is available through industry and through other governmental sources such as the National Science Foundation or the US Environmental Protection Agency. University researchers are also strong local resources for public outreach and education related to greenhouse gas generation and storage.
Challenges in Academia
The foremost challenge is dwindling research funding, not just for gas flooding but any oil- and gas-related research. When oil prices drop, “research” becomes a bad word among all funding sources. This needs to change. Second, university research should continue to focus on the free flow of knowledge without boundaries, open-minded examination of new concepts and ideas, and independent pursuits of new realms of science. Third, professional journals should publish new material even in the face of controversy—academic careers depend on publications. Fourth, the industry that benefits from these resources should actively participate in university research for it to be relevant. Operators should be more open to implement pilots to try out new ideas, irrespective of their origin, be it the industrial labs or academia.
References
Christianson et. al., SPE 71203, 1998
Rao et al., SPE 89357, 2004
![]() | Dandina N. Rao is Emmett Wells distinguished professor in the Craft and Hawkins Department of Petroleum Engineering at Louisiana State University (LSU). He holds a BTech with Distinction from India, an MS from the University of Saskatchewan, and a PhD from the University of Calgary. After 17 years of research and development work (Shell Canada, Petroleum Recovery Institute, and BDM Petroleum Technologies), he came to LSU in January1999. His experimental research interests include reservoir condition rock-fluids interactions and thermal and nonthermal EOR. |
![]() | Richard G. Hughes is a professional-in-residence for the Craft and Hawkins Department of Petroleum Engineering at LSU. He earned a BS in petroleum engineering from New Mexico Institute of Mining and Technology and held various production, reservoir, and information technology positions with Tenneco Oil, Dwights Energy Data, and Amerada Hess. He then obtained his MS and PhD in petroleum engineering from Stanford University before joining the University of Oklahoma as an assistant professor. His research interests include utilizing CO2 for EOR and carbon sequestration, modeling of multiphase flow in porous media and rock fractures, reservoir surveillance, production data analysis, and unconventional field reservoir engineering. |