To meet ambitious emissions-reduction targets, the UK must envision dramatic changes to the energy economy. Two technologies that may play a part in the decarbonization of the UK energy business are carbon capture, use, and storage and the use of hydrogen as an energy carrier and energy storer. This approach builds on the UK’s gas-network infrastructure, which can be repurposed to avoid becoming stranded and to avoid the enormous expense of increasing the capacity of the electricity-transmission network. The complete paper explores the ways in which northeast Scotland can best integrate its infrastructure into this challenge, with emphasis on the unfolding ACORN carbon capture and storage project.
Introduction
At its core, the intent of Acorn is to develop a hub for carbon capture and storage (CCS), enabling a more sustainable energy system.
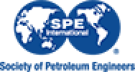