Over the years, hybrid systems have been installed in horizontal wellbores to increase the number of compartmental sections for hydraulic fracturing because of the limitations of ball-actuated fracture sleeves. Experimenting with hybrid systems provides operators with the ability to optimize spacing of fracture stages along the horizontal section when sleeve technology alone does not allow for the desired number of stages. The costs are higher for operators to perform a hybrid-type completion, however, and this has driven enhanced sleeve technology to allow for all-sleeve completions.
Introduction
The Bakken shale located in the Williston basin covers an area that includes portions of North Dakota and Montana in the US and Manitoba and Saskatchewan in Canada (Fig. 1). An unconventional reservoir, the Bakken formation is one of the last giants to be discovered in North America. The US Geological Service estimates that the undiscovered US portion of the Bakken formation holds 3.65 billion bbl of oil, 1.85 Tcf of associated gas, and 148 million bbl of natural-gas liquids.
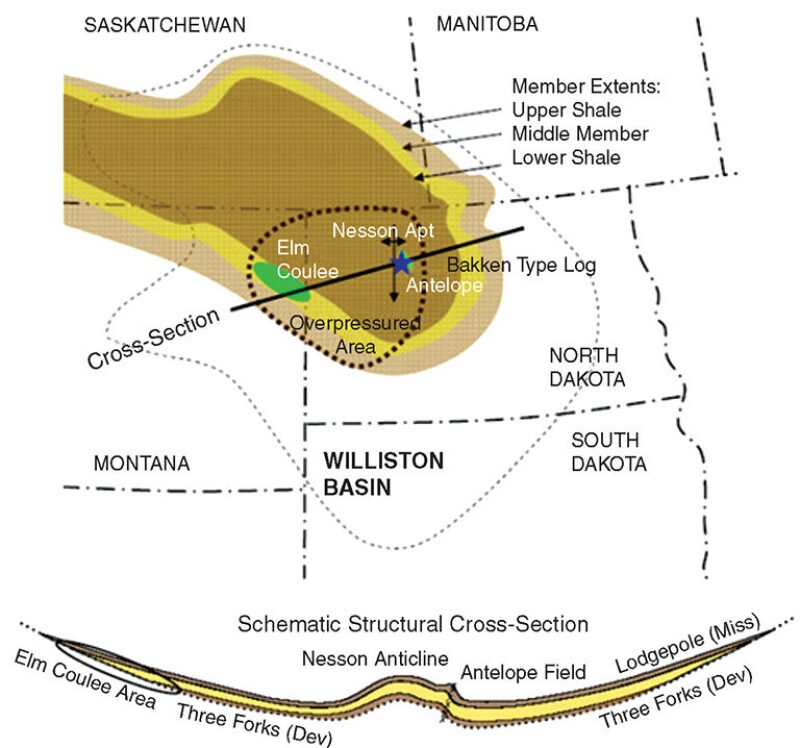
Advances in horizontal drilling of extended-reach wells and in completion techniques have increased the amount of recoverable oil and gas. Wells drilled in the Bakken are typically drilled horizontally across two 640-acre sections, with laterals that can extend more than 9,000 ft. Two completion methods known for cost-effectiveness and high well efficiency that are used in these wells are the openhole-packer system and the sleeve one-trip system.
Openhole-Packer and Sleeve Completions
Openhole completions performed in the Bakken are commonly run as a one-trip system on drillpipe containing the following equipment: a hydraulic or mechanical running tool, liner-top packer or liner-hanger system, openhole packers, fracture sleeves, and float equipment. Once the system is in position, the appropriate fluid is displaced, and the liner-top-packer is set; the running tool is disconnected from the system and removed from the well. The openhole packers provide compartmental isolation along the horizontal wellbore with fracture sleeves placed at each stage, forming a pathway for stimulation fluid and the production of hydrocarbons.
When the pumping equipment is mobilized, a series of balls is dropped (in order of smallest to largest) that will land on corresponding ball seats starting at the toe of the well, isolating previously fractured sections and shifting open the fracture sleeves. After the pumping is completed, the well can be placed on production. During the production phase, the balls that were pumped down the wellbore and used to open the fracture sleeves will flow off the ball seats and back up the wellbore.
Components placed in the open hole are specifically developed for multistage applications. The openhole packers are manufactured with a swellable elastomer, which reacts to oil-based fluids (diesel) and expands out to the wellbore, creating zonal isolation.
Fracture sleeves contain specifically designed ball seats that are pinned closed using shear screws when installed in the well. Through the use of fracture balls, a differential pressure is created across the ball seat, allowing for an increase in tubing pressure that shears the shear screws, shifting the sleeves open to expose the fracture ports. The pump rates and types of fracture fluid need to be known before the fracture sleeves are installed to allow for the correct number of shear pins to be placed in the sleeves. A differential pressure is created across the seats from the high flow rates in the wellbore. In some cases, the smaller-internal-diameter fracture sleeves placed closest to the toe of the well will require a higher actuation pressure than those placed toward the heel of the well to compensate for the forces created during the fracture treatments.
The positive impact realized from a faster hydraulic-fracture process is a localized wellsite benefit. On a larger scale, the operator realizes monetary benefits as well. The wellsite impact from spending fewer days on location during the hydraulic fracturing is considered from both a health, safety, and environmental standpoint and an operational-risk and cost-reduction standpoint. A shorter fracture time minimizes disturbances to local populations and lowers the amount of CO2 emissions. The operator experiences more-effective use of company personnel.
Fracture sleeves have proved to be reliable and conserve water and time between fracture stages. The cycle time between wells and the additional number of wells that can be completed from the reduced number of days spent on location allow operators to increase production across the field by bringing more wells on line.
Recent Advancements in Technology
In 2007, a new packer technology was combined with existing fracture-sleeve capabilities to provide the necessary components for a multistage, one-trip completion in the Sanish field. This new packer technology was a swellable elastomer wrapped and bonded to casing joints. Depending on the choice in elastomer, the reaction will occur in either oil-or water-based solutions. It is common in the Williston basin to use elastomers that react with oil to swell and create annular seals.
The initial fracture-sleeve application was deployed in November 2007. Upon reaching the target setting depth with the completion, diesel was displaced along the horizontal section containing the openhole packers. Behind the diesel, a ball was pumped to a ball seat for setting the liner-top packer and releasing the running tool from the bottomhole assembly. With the completion in place, the rig was moved from location and preparations for hydraulic fracturing began. Downhole temperature for this well was 210°F, requiring 3 days for the packers to form a seal against the formation.
The hydraulic-fracturing operations began by opening the pressure sleeve. A pressure of 4,000 psi was applied to shift the pressure sleeve. The first stage of the fracture was pumped through this sleeve. The remaining seven stages were pumped through ball-actuated fracture sleeves. The entire fracturing treatment was completed in less than 12 hours. Fracture specifics included the use of 1,800,000 lbm of natural-sand proppant and 18,000 bbl of water pumped at rates of 30 to 40 bbl/min.
The initial production for this well was 1,323 bbl of oil and 2 MMcf of gas during a 24-hour flow test. During the next 30 days, the well averaged 818 B/D of oil production and 828 Mcf/D of gas production. In the winter months, the use of conventional perforating guns and composite plugs would have caused the completion process to take a minimum of 4 days.
Throughout 2008, the number of stages in the one-trip openhole-packer-and-sleeve completions increased to 10. Engineers realized that production could be increased if the spacing between sleeves/packers (stages) could be reduced. In 2009, this was accomplished by creating and deploying hybrid completions consisting of two technologies: the use of fracture sleeves for the toe section of the wellbore and the use of composite fracture plugs for isolation and perforating guns for communicating with the formation during the remaining stages (Fig. 2). The installation of the hybrid completion was relatively unchanged. For the sections of the wellbore that were to use a plug-and-perforation method, openhole packers were spaced out with casing joints. The operator would be able to benefit from the fracture-sleeve efficiencies during the first 10 stages, but would then need to pump down composite fracture plugs and perforate each section for the remaining stages.
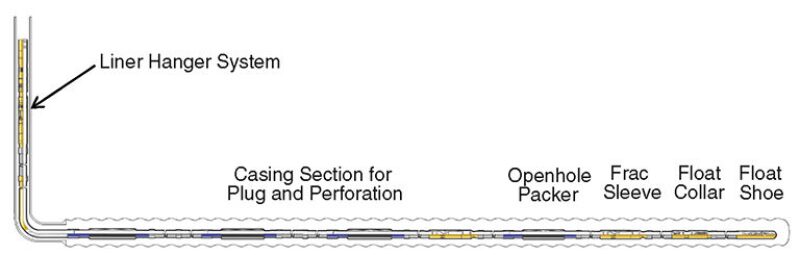
In October 2009, new fracture-sleeve technology was deployed that increased the number of stages in a one-trip openhole-packer/sleeve system to 24. In 2010, the hybrid-system approach was expanded to include more stages as the optimal spacing in different parts of the Sanish field was evaluated. During this time period, additional components of the wellbore evolved with industry-adopted pressure-integrity-verification requirements and with longer-lateral drilling. Liner-top-packer- and liner-hanger-system choices are reviewed on the basis of vertical or horizontal tool settings, pressure-rating requirements (pressure-integrity-test requirements), and combined loading of installed equipment during hydraulic-fracturing operations. Software models are generated, and separate calculations are performed to determine resultant loading conditions from pressure-testing and hydraulic-fracturing operations.
Recloseable Fracture Sleeves. These sleeves have been designed to shift open and lock into the open position. During the life cycle of an unconventional reservoir, there are opportunities to refracture complete wells or wellbore sections to re-establish production. In June 2009, a packer/sleeve completion system was installed with recloseable fracture sleeves. The proposal included hydraulically fracturing the well to produce hydrocarbons before returning to the well for in-field refracture development. The ball seats would need to be milled out before shifting the fracture sleeve to the closed position and isolating the fracture ports. This would provide the full-bore internal diameters required to install composite fracture plugs and refracture each section by use of the plug-and-perforation method. Since the installation, additional information has been gained in regard to required spacing between compartments, and the refracturing has not occurred.
Monitoring Multistage Fracturing
Proper spacing of the compartments created by openhole packers along the horizontal section is determined through analytical methods. One such method uses microseismic tools, where geophone arrays monitor shear failure along pre-existing natural fractures on the basis of acoustic signatures. Monitoring of the fracture propagation provides data that can be used to verify decisions made regarding spacing changes between compartments and wells across lease sections (Fig. 3). A more-complex configuration, which provides additional data regarding the reservoir and hydraulic fracturing, includes the use of downhole flow and temperature sensing. This type of monitoring can be achieved with distributed-temperature-sensing (DTS) or permanent-downhole-monitoring (PDHM) systems, which are either electrical- or fiber-optic-based. In April 2010, a 10‑stage openhole-packer/sleeve system was installed with DTS and PDHM systems. Afterward, pressures and temperatures were monitored during the fracture of surrounding wellbores, real-time monitoring occurred during the fracturing, and post-fracture monitoring was available to validate the performance of the openhole systems, providing data needed for drilling plans in the Bakken and Three Forks formations. Other methods applied during field-development planning include drillstem testing, core analysis, and implementation of logs to characterize the reservoir.
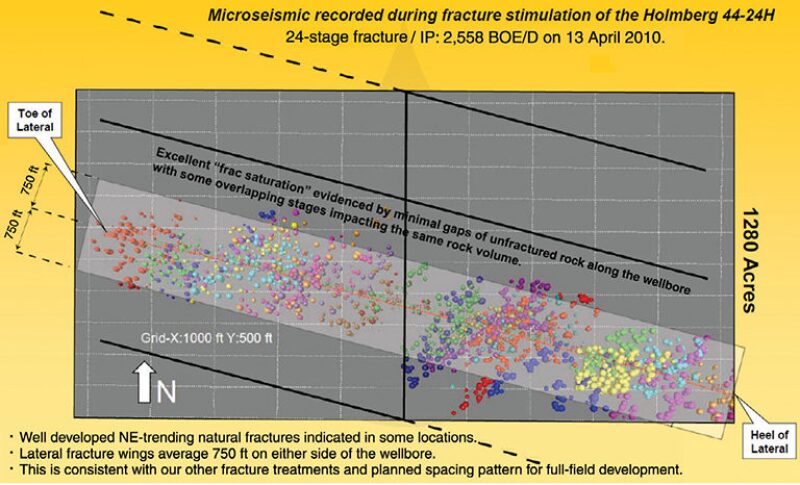
This article, written by JPT Technology Editor Chris Carpenter, contains highlights of paper SPE 159586, “Advancements in Openhole-Completion Technology Increase Efficiencies and Production in the Williston Basin,” by John Paneitz, Whiting Petroleum, and C. Christopher Johnson, Matthew White, and George Gentry, Baker Hughes, prepared for the 2012 SPE Annual Technical Conference and Exhibition, San Antonio, Texas, USA, 8–10 October. The paper has not been peer reviewed.