Are fugitive releases of natural gas and flaring environmental concerns? Can these be ameliorated today and even better in the future? Yes and yes.
A paper in 2011 by Howarth et al. suggested that fugitive methane emissions (releases directly to the atmosphere) from shale gas production amounted to 3.6% to 7.9% of all gas recovered and that those numbers were worse than coal. The authors took the comparison of greenhouse gas emissions to the precombustion stage. Since the dominant use of coal is for electricity production, post-combustion comparisons are merited. In so doing, natural gas is favored because power plants using natural gas are up to about 50% more efficient on conversion than coal plants.
Many of these original assumptions have been challenged and the data verified, most recently by an ongoing study by Allen et al. in a 2013 report in the Proceedings of the National Academy of Sciences. This Environmental Defense Fund-sponsored study reports that 0.42% of “the emissions are released” at the production site. The study is ongoing and more clarity is needed, especially regarding the locations and amounts of the releases. Since this and other studies were almost a direct consequence of the Howarth paper, much good came of that despite the justifiably disputed elements of the original work.
The principal reason for environmental concern about releases of methane is that it is a more powerful greenhouse gas than carbon dioxide (CO2). The potency numbers are somewhat in dispute and the general public may be confused. There is general agreement with the belief that methane is about 25 times more potent than CO2. The accompanying sidebar gives the science behind the calculation and explains why some believe it to be 72 times more potent.
The oil and gas industry continues to take steps to minimize methane release. Methane in the water flowing back from the well is usually separated, so collection is not an issue. If a pipeline is available, it is utilized. In the early days of a prospect there may not be an export line. In these cases, at the very least, the gas ought to be flared, thus reducing the potency of the released gas.
The inadvertent release of methane can come from hatches, gaskets, and the like that are not properly sealing. Also, in the distribution system, some valve systems operate using natural gas as the actuating fluid, and some release occurs each time they are operated. Alternative valve mechanisms are available, using compressed air, for example. Leaks are currently measured using infrared cameras. The ARPA-E Methane Observation Networks with Innovative Technology to Obtain Reductions (MONITOR) program is uniquely targeting innovative means for identifying methane leaks on the rigsite from a variety of sources. All of the foregoing ought to underline the fact that the industry is now fully aware of the issue and is getting its collective arms around solving the matter. Solutions are in the economic interests of the operators: gas that leaks away is gas that does not get sold.
Global Warming Potential of MethaneThe mechanism by which methane warms the Earth is similar to that of CO2. Infrared radiation attempting to escape into space is absorbed, and thus causes the net warming of the Earth. |
Flaring of Associated Gas
When oil is produced, there usually is natural gas associated with it. This is because all oil was formed from the action of pressure and temperature on organic matter a couple of hundred million years ago. The early immature form of this matter is known as kerogen. Continued thermal maturation converts it first to oil and finally to gas. At any given time if oil is the predominant fluid, chances are some part of it matured all the way to gas. This, then, is the “associated gas.” The proportions are greater when the oil is light, as in the case of shale oil. Incidentally, the same holds if the predominant fluid is gas. Some fraction could be relatively immature, with oil-like larger molecules like propane and butane. These are known as natural gas liquids (NGLs). Much of the shale gas production has this associated liquid. When it substantially does not, because of a high state of thermal maturity, it is known as “dry” gas. The Haynesville is one such play.
Associated gas is often economically or logistically stranded. Gas pipelines may not exist because of the low flow volumes forecast or the sheer distance. In the latter category are offshore platforms, especially off the west coast of Africa. In areas such as the Bakken, it could be a matter of timing; the pipelines are slow to arrive. In many cases the volumes are too low to support export lines. In any case, the world today flares in the vicinity of 5 Tcf/year. North Dakota today flares about a third of the associated gas, which amounts to about 0.13 Tcf/year. Even at today’s depressed gas prices, that amounts to more than USD 350 million in economic loss, not to mention the environmental impact of the CO2.
Monetizing Low Volume Associated Gas
There is a truism in chemical processing: Size matters. Bigger is better for the economies of scale. Consequently, conventional processes fail to address the unique needs of low volume natural gas to be converted to something movable and saleable. So, how low are these volumes involved? To estimate this we investigated the distribution of flared gas in North Dakota and the results are shown in Fig. 1.
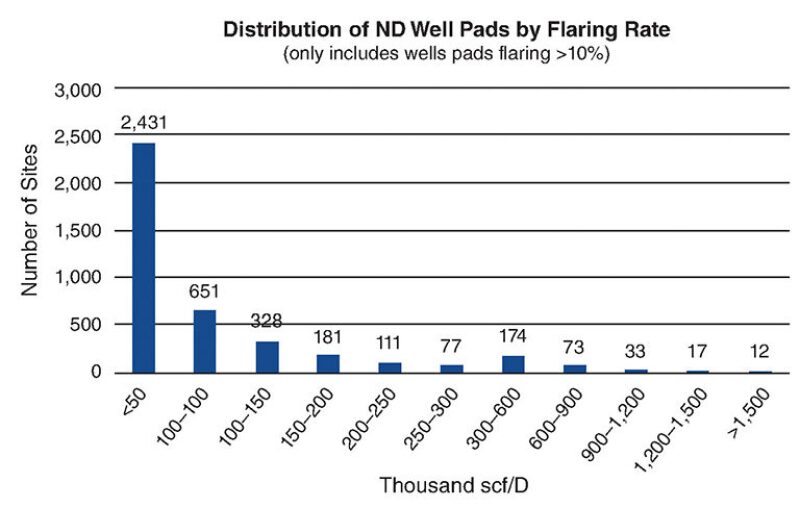
Of note is that the majority of flared gas is from pads producing as little as less than 50 Mcf/D (50,000 cubic feet per day) to 200 Mcf/D. An ARPA-E funded project (2012) is in development to target feeds of 50–300 Mcf/D to convert the associated gas to methanol. The process is designed to all be housed on two flatbed trucks. This will allow the unit to be moved to different locations. This and similar developments (World Bank 2014) could also service other stranded gas sources such as those described earlier at shale gas production locations. Any new processes must take into consideration the fact that the associated gas is not pure methane. It will typically have ethane, propane, and butane to several percent. Even flaring equipment has to be adjusted to ensure complete combustion of these diverse gases.
Two other recent developments could target this opportunity. One is efficient compression of the gas to where the compressed gas can be transported to a central location for processing or transmittal. Another is known as mini-liquefied natural gas (LNG) in which the gas is liquefied and then transported. Here, too, the technological advance is that conventional LNG plants are up to 100 times larger than these units. This class of solutions is sometimes collectively referred to as virtual pipelines.
Other Manmade Sources of Methane
Farm animals classified as ruminants are large sources of methane. In the US, this source is estimated to account for emissions on the same scale as from the oil and gas industry. Cattle in particular are the largest single source. The grass they eat is inefficiently converted to useful energy in the animal. Some of it reacts with resident bacteria to yield methane. This is expelled from both ends of the animal: flatulence as well as burping. The most promising avenue for amelioration is diet modification such as the addition of lipids to the feed (Martin et al. 2007). Similar to the motivation of oil and gas operators to use the fugitive gas, here too the farmer would benefit because more of the feed would go to make meat or milk. However, the remedies proposed above for the oil industry will not easily apply here.
The third-largest source of fugitive methane is landfills together with animal waste impoundments such as swine lagoons. These are fairly well-suited to utilize the small footprint, gas-conversion technologies mentioned earlier. This gas source will not have the larger molecules such as propane. But it may have contaminants such as CO2 and possibly sulfur-bearing gases. The processes would have to account for these. This is technically feasible.
In summation, emissions of manmade greenhouse gases, in particular fugitive methane and CO2 from gas flaring, are environmental concerns. Active steps are currently being taken to ameliorate the effects. Ongoing innovation in this space is likely to yield significant results and ought to be encouraged.
References
- Howarth, R.W., Santoro, R., and Ingraffea, A. 2011. Methane and the Greenhouse-Gas Footprint of Natural Gas From Shale Formation. Climatic Change, (retrieved 14 March 2012).
- Allen, D.T., Torres V.M., Thomas, J. et al. 2013. Measurements of Methane Emissions at Natural Gas Production Sites in the United States, PNAS, 110 (44): 17768–17773.
- ARPA-E. 2012. Compact Inexpensive Reformers for Natural Gas (28 November 2012), (accessed 30 January 2016).
- World Bank. 2014. Associated Gas Monetization via Mini GTL: Conversion of Flared Gas Into Liquid Fuels and Chemicals, April 2014 Update.
- Martin C., Ferlay A., Chilliard Y., et al. 2007. Rumen Methanogenesis of Dairy Cows in Response to Increasing Levels of Dietary Extruded Linseeds. 2nd International Symposium on Energy and Protein Metabolism and Nutrition, Vichy, France, 9–13 September.
Rao also advises the nonprofit RTI International, venture capitalist Energy Ventures, and firms BioLargo, Global Energy Talent, Biota Technology, and Eastman Chemicals. He retired as senior vice president and chief technology officer of Halliburton in 2008 and followed his wife to Chapel Hill, North Carolina, where she is on the University of North Carolina faculty. Later that year he took his current position. He also is past chairman of the North Carolina Mining and Energy Commission.
Rao’s book Shale Gas: the Promise and the Peril was released in 2012 by RTI Press and can be found at www.rti.org/shalegasbook. It is written for general audiences and is intended to inform the debate about on fracturing for shale gas. The revised edition with six new chapters and extensive revision was released in August 2015 (www.rti.org/shaleoilandgas).
Rao holds a bachelor’s degree in engineering from the Indian Institute of Technology in Madras, India, along with a master’s degree and a doctorate in materials science and engineering from Stanford University. He is the author of more than 30 publications and has been awarded 40 US patents and foreign analogs.