Excessive water production from hydrocarbon-producing wells can adversely affect the economic life of the well. The major challenge for water control in carbonate reservoirs is polymer bonding to the rock surface. Most commercial products are designed for sandstone formations, and most polymers will not strongly adsorb to carbonate reservoirs. A new water-shutoff polymer system has been developed for carbonate formations and shows great stability.
Introduction
The objective of the project was to develop additives to be used as a smart sealant that can be used to control unwanted water production.
×
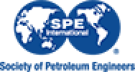
Continue Reading with SPE Membership
SPE Members: Please sign in at the top of the page for access to this member-exclusive content. If you are not a member and you find JPT content valuable, we encourage you to become a part of the SPE member community to gain full access.