Because of current oil and gas industry economics, evaluating the return on investment for any well-intervention campaign is crucial, as is applying an assurance process to help quantify desired production improvement. This paper presents the planning and execution of a matrix-stimulation pilot project in the heavy-oil Chichimene Field in Colombia. The approach is based primarily on a work flow that includes characterizing formation damage, reviewing laboratory tests, validating well selection, and determining economically viable placement and diversion techniques.
Damage Mechanisms
Heavy-oil reservoirs are prone to almost every formation-damage mechanism known. Damage mechanisms encountered include fines migration, paraffin and asphaltene deposition, various forms of scale, and clay swelling.
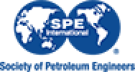