Matrix acidizing is commonly used as a preflush to the hydraulic-fracturing stimulation of shale formations. The process dissolves sediments and mud solids that inhibit the permeability of the rock, enlarging the natural pores of the reservoir and stimulating flow of hydrocarbons. In this paper, the mineralogical and physical responses to matrix acidizing of several important North American shale formations are evaluated.
Introduction
A few studies have quantified the effect of hydrochloric acid (HCl) matrix acidizing on mineralogical and physical properties of shale formations. However, less is known about the development of conductivity and the acid concentrations necessary to optimize conductivity and, by extension, the impact on production and rock stability. The mechanical properties of shale formations vary as a function of mineralogy, with shales rich in brittle minerals such as quartz and carbonates having higher Young’s-modulus values. Increases in the clay content, total organic carbon (TOC) content, and porosity may lower the Young’s-modulus values. Therefore, dissolution of brittle minerals, while improving short-term conductivity, may lower long-term shale rock stability. HCl reacts rapidly with calcite [Ca2(CO3)2] and, to a lesser extent, with dolomite [CaMg(CO3)2], and it is predicted that the main impact of HCl on shale will vary as a function of how much calcite is dissolving, which, in turn, is controlled by acid strength, temperature, and pressure.
Shale Samples
Eagle Ford shale reservoir core samples and outcrop samples from the Mancos, Barnett, and Marcellus shale formations with contrasting assemblages—ranging from the calcite-/clay-rich, quartz-poor Eagle Ford to the quartz-/illite-rich, carbonate-poor Mancos—were used in this study.
The Eagle Ford shale contains the greatest proportion of carbonate, but the absolute volumes of carbonate vary systematically. At deeper structural levels, such as those exploited in south Texas, there is upward of 70% carbonate by volume. With progression toward the northwest, the clay content increases, and the formation is exploitable at shallower depths. The high percentage of carbonate makes it more brittle and fracturable.
The Mancos is predominately steel-gray sandy shale but includes stringers of earthy coal, impure limestones, and many thin beds of fine-grained yellow and brown sandstone that are chiefly composed of subangular and angular quartz grains cemented by lime.
The Barnett is a very brittle gas-bearing siltstone. Most Barnett shales are siliceous mudstones, rich in quartz, and may be considered argillaceous siltstones. Some of the Barnett lithofacies are insensitive to acid because of low volumes of carbonate, but their clay-mineral assemblage may be moderately sensitive to fresh water. Other lithofacies have higher abundances of carbonate and are therefore more reactive to matrix acidizing.
The Marcellus formation is dominated by black shale with some interspersed limestone beds. Bedding is well developed and often splits along bedding planes. Pyrite is also relatively common in this shale.
Porosity and Mass-Loss Measurements of Shale Samples
Eagle Ford core samples were dried before removing reservoir in-situ fluids by toluene extraction before all experiments. Air-saturated core samples of individual shales measuring 2.54 and 3.81 cm in diameter and 0.76 to 5.08 cm in length were characterized by computed-tomography (CT) scanning. The cores were saturated with oil for 2 weeks, excess oil was removed under vacuum for 2 days, and the oil-saturated samples were reimaged by CT scanning to calculate preacid-treatment porosity.
A second set of samples were weighed as dry and then placed in 1-wt% active, 2-wt% active, and 3-wt% active HCl solutions at 200°F for 10, 20, 30, and 180 minutes. The acid solutions used to treat Mancos samples were mixed with 30‑wt% (equivalent) sodium chloride (NaCl) to prevent clay swelling. 5-wt% (equivalent) NaCl was used to prevent clay swelling in the remaining experiments for the other rocks. All samples were dried after the acid treatment and reweighed to calculate mass loss. Post-acid-treatment porosity was then calculated.
For a discussion of X-ray-diffraction studies and Rietveld refinement procedures for these samples, please see the complete paper.
Scanning Electron Microscopy and Energy Dispersive Spectrometry
Bedding-perpendicular thick sections of selected core plugs were prepared and polished with 1200 alumina grit. The thick sections were carbon coated and placed in a scanning electron microscope with a spectrometer. 2D element-distribution maps were collected for selected areas of each thick section with an electron beam. Element-distribution maps were collected, and from each area of interest a minimum of 16 frames were collected and the results automatically compiled to produce a single X-ray map that was corrected for beam drift over the course of the acquisition time (approximately 8 hours).
Mechanical-Property Measurements
The Eagle Ford samples have the highest calcite abundance among the shale rocks and were therefore predicted to be the most susceptible to changes in mechanical properties as a function of exposure to HCl; thus, they were the focus of mechanical-property studies. A new suite of Eagle Ford shale samples measuring 1.905 cm in diameter and 3.81–4.76 cm in length were exposed to the matrix-acidizing experiments (1-, 2-, and 3-wt% HCl plus 5% NaCl) and used to determine compressive strength (Fig. 1 above). In addition to measuring untreated and acid-treated shales, a sample of Eagle Ford treated with 5-wt% NaCl was tested. The matrix-acidizing experiments were performed at 200°F until the effervescence of the sample ceased. The 5-wt%-NaCl-solution sample was also treated at 200°F for the same time duration as the matrix-acidizing experiments.
Data Analysis and Discussion
Effect of HCl on Shale Mineralogy. Phase identification and Rietveld refinement of X-ray-diffraction patterns were used to identify the major rock-forming phases and provide semiquantitative abundances for each sample. Comparison of pre- and post-HCl-treatment patterns shows that, in most cases, some calcite (and occasionally dolomite) was dissolved in the samples. However, Rietveld refinement studies were unable to reconcile and quantify the changes in relative mineral proportions on the basis of the mass-loss experiments.
For Eagle Ford samples, Rietveld refinement of the primary sample gives a close match to published mineralogy, and a measureable decrease in the intensity of the 104-calcite peak (100% relative intensity) in the post-acid-treatment sample is observed. The Mancos pre-HCl-treatment mineralogy is also in good agreement with published values. However, there is an increase in the intensity of the 104-calcite peak in the post-HCl-treatment sample. The experimental observations and the results of mass-loss calculations suggest that carbonates were dissolved during acidizing experiments; therefore, the observed increase in relative peak intensity is attributed to preferred orientation effects in the X-ray-diffraction pattern that could not be resolved by Rietveld refinement. Calcite was not positively identified in Barnett samples, and, while dolomite was identified on the basis of peak interpretation, pattern matching was unable to quantify its abundance accurately. For Marcellus samples, Rietveld refinement of the pre-HCl-treatment sample shows a good match with published data, although measurable differences between pre- and post-HCl-treatment samples could not be resolved.
The results of bulk-rock compositional analyses show a degree of compositional heterogeneity at the submillimeter bedding scale. The Eagle Ford pre-HCl-treatment sample was exceptionally rich in calcium oxide (CaO) in analyzed areas, and, with the low phosphorus pentoxide content, it is predicted that the calcium budget is held in carbonate. However, in the post-HCl-treatment sample, the mapped area has a lower silicon dioxide (SiO2) content compared with pre-HCl-treatment areas mapped, demonstrating the tremendous complexity of these samples; HCl treatment should not have impacted SiO2 abundance and distribution. The compositional analyses and element-distribution maps demonstrate that the majority of calcium is hosted by carbonate, probably calcite, with very little contribution from apatite or other calcic ferromagnesium phases.
For Mancos samples, studies of the post-HCl-treatment sample show very comparable analysis, with differences between measured bulk-rock compositions within the analytical error of the instrument.
On the other hand, in the Barnett samples, there are decreases in the calcium and phosphorus abundances that appear correlated with one another, suggesting that dissolution of minerals during acid treatment did occur—but it was phosphate being dissolved, not carbonate. Marcellus samples showed the same heterogeneity in the tested samples; the pre-HCl-treatment samples showed higher CaO compared with the post-acid-treatment sample and less SiO2.
Effect of Contact Time on Shale Porosity and Mass Loss
Mass loss for the samples shows a correlation with mineralogy and average post-porosity, with the Eagle Ford samples (with higher carbonate abundance) showing the greatest mass loss. The degree of carbonate dissolution in Eagle Ford systematically increases with increasing acid concentration and contact time. Eagle Ford samples lost between 1 and 16% of their mass, while their porosities increased from 1.2 to 8.7%. The nonsystematic trend of mass loss between the 20- and 30-minute experiments in 2-wt% HCl is attributed to the variations in mineralogy (heterogeneity) between the tested samples at scales comparable with those identified in the compositional analyses.
In the Mancos, Barnett, and Marcellus samples, the mass-loss correlation with the contact time is similar to that of the Eagle Ford samples, but all measured variables are lower. This is proposed to be a consequence of the much lower relative abundances of calcite (and other carbonates) in these shales. Measured porosities in post-acid-treatment samples do not have a linear correlation with mass loss, contact time, or acid strength. It is proposed that this is because the changes in porosity are dominated by crack development and not carbonate dissolution, which results in enhancement in porosity on the scale of 2.7–19% for Mancos samples, 7.5–16.5% for Barnett samples, and 2.2–7.28% for Marcellus samples.
Effect of HCl on Eagle Ford Shale Mechanical Properties
Characteristics of the stress/strain curves for Eagle Ford shale samples are compared after the different HCl concentrations and as a function of 5% NaCl solution (Fig. 2). In the nonacid-treated sample, a single crack propagated in a diagonal manner neither parallel nor perpendicular to bedding planes, while in the 5-wt%-NaCl-treated sample, cracking and fracture development was a multistage process (Fig. 2 and Figs. 3a and 3b). Each stage of cracking for 5-wt% NaCl represented a new layer crack, which may imply that NaCl affects each layer of the sample differently depending on the clay percentage (Fig. 2).
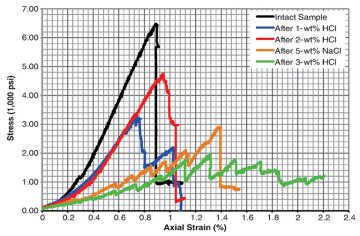
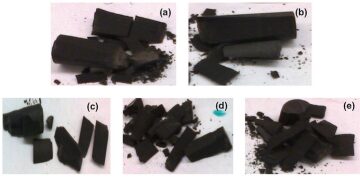
Samples of Eagle Ford treated with 1-wt% HCl+5-wt% NaCl cracked and fractured through an intermediate mechanism compared with the untreated and 5-wt%-NaCl-treated sample. In that experiment, two cracks developed that could represent two layers breaking at different stresses, depending on how HCl affected each layer, which would be controlled by mineralogy (Figs. 2 and 3c). The sample treated with 2-wt% HCl+5‑wt% NaCl behaves similarly to the untreated sample, with only one crack developing; the sample treated with 3-wt% HCl+5-wt% NaCl behaved similarly to the 5-wt%-NaCl-treated sample, where different cracks represent different layers breaking at different stresses. These results are difficult to interpret because they do not appear to correlate with each other. In the case of the 2-wt%-HCl+5‑wt%-NaCl-treated sample, it is proposed that the sample’s behavior may be the result of contrasting modal mineral abundances between the studied samples. Meanwhile, the more-complex distribution/development of cracks in the 3-wt%-HCl+5-wt%-NaCl experiment may be a consequence of heterogeneous crack and fracture distribution attributed to highly variable calcite distribution between bedding lamina. The measured Young’s modulus and unaxial compressive strength of the untreated Eagle Ford sample were in good agreement with published data (please see the complete paper for these specific data).
This article, written by JPT Technology Editor Chris Carpenter, contains highlights of paper SPE 165689, “Effect of Low-Concentration Hydrochloric Acid on the Mineralogical, Mechanical, and Physical Properties of Shale Rocks,” by Samiha Morsy, SPE, C.J. Hetherington, and J.J. Sheng, Texas Tech University, prepared for the 2013 SPE Eastern Regional Meeting, Pittsburgh, Pennsylvania, USA, 20–22 August. The paper has not been peer reviewed.