The recycling of produced water and fracture flowback water for reuse in hydraulic fracturing is on the rise in the development of unconventional resource plays. The factors driving the conservation of water are the limitations in sources of fresh water in areas with a high rate of development, the attractive economics of recycling compared with tanker truck transportation costs, minimization of road traffic to reduce environmental impacts, and water disposal costs.
Fracture fluid, which ranges from water to slickwater and gels, requires fresh water as a makeup fluid to achieve consistency in the composition of the fracturing fluid and optimal fracture results. Traditional sources of fresh water for hydraulic fracturing include glacial and bedrock aquifer systems, surface waters, and municipal supplies.
Economics of Conservation Gain Favor
In its April 2010 report, “Bakken Water Opportunities Assessment—Phase 1,” the University of North Dakota’s Energy and Environmental Research Center (EERC) cited current water-handling costs in the Bakken (Table 1) as reported by several producers in the state. The report pointed to a strong incentive of cost reduction for consideration of alternatives to the continued use of fresh water.
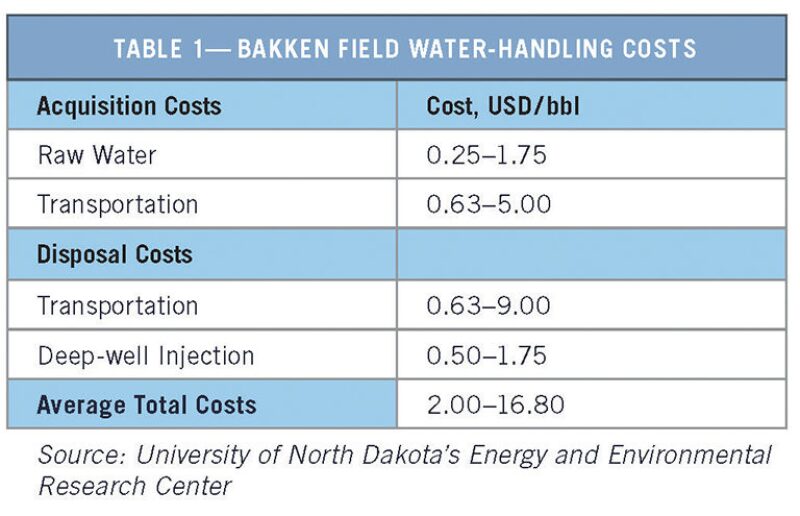
The volume of water used in a Bakken play fracture ranges from approximately 0.5 million to 3 million gallons (10,000 bbl to 60,000 bbl), depending on the number of stages in the fracture, with multistage fractures increasingly being used for improved fracture results. In 2011, Baker Hughes completed a 40-stage openhole completion in the Bakken’s Williston Basin.
The cost to purchase raw water for use in the Bakken play ranged from USD 0.25/bbl up to USD 1.75/bbl, while the cost of transporting that water to the fracture site ranged from USD 0.63/bbl up to about USD 5/bbl.
Transportation costs, the most variable factor in water acquisition costs, depended on several factors, including trucking charges, wait time, and hauling distances. Trucking charges, including wait time, were reported to range between USD 110/hour and USD 150/hour.
Costs for deep well injection ranged from USD 0.50/bbl to USD 1.75/bbl. Transportation costs accounted for the most significant cost associated with disposal.
The average total cost for handling water ranged from USD 2/bbl to USD16.80/bbl. According to the report, transportation costs represent from 56% to 84% of the total water-handling costs, thus justifying the assessment of the economic potential of fracture flowback water recycling and reuse.
The EERC report addressed only the Bakken development’s water-handling variables and costs. However, other unconventional plays face their own challenges, and producers and oilfield service companies have found unique solutions in processes, infrastructure, and facilities.
Pinedale Anticline Field
Located in southwestern Wyoming, the field is an example of where recycling water has contributed significantly to water supplies for field development. The need to conserve water and limitations on disposal injection led to early consideration and implementation of water recycling processes.
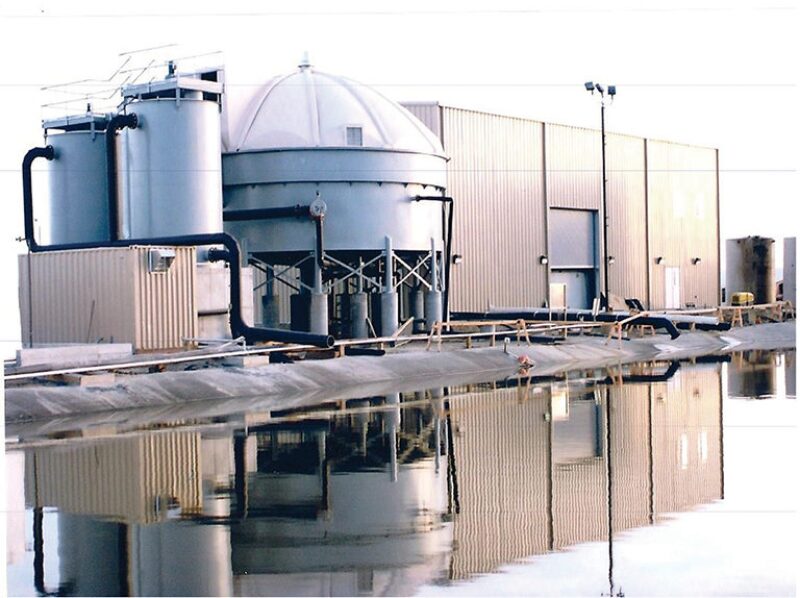
Production from the field comes from tight gas sands, where as many as 15 to 30 completion stages may be individually fractured for a total well completion. The fracturing process operates 24 hours per day over three to four days for a single well. Fracturing water load volume is approximately 30,000 bbl with initial load recovery of 25% to 50% (Shafer 2011).
Lee Shafer, president of Integrity Production Services, said there was no processing of fracture water in the Pinedale Anticline in 2004. Produced water and fracture flowback was trucked away and disposed into injection wells or collected in evaporation ponds.
Operators later began using in-field recycling with clean produced water for fracturing with some success; however, this required significant resources to stage the water at sufficient rates for the 24-hour field operations. To take the recycling a step farther, the flowback water required the processing of varying mixtures of residual guar gels, polyacrylamide residue, a variety of spent fracturing additives, and significant levels of suspended solids.
Anticline Disposal began recycling produced and flowback water in the Pinedale in 2006. Its facility treats produced and flowback water and sends it back to the operators for future field development uses, such as fracture water. The drilling companies tap onto Anticline Disposal’s high-pressure pipeline to deliver fracture water directly to their work sites.
Shafer said, “The process was designed to fit a specific niche—to recycle as much water as possible to the local fracturing market and then to process the surplus water.
“Our assessment of water-handling projects begins with looking at the cost of aggregating (trucking or gathering system), processing, and storing the water. The economics for collecting the water and the redistribution of the processed water to the successive fracturing jobs with buried pipe or surface line are critical.”
The Pinedale process was designed and constructed to meet the field development needs in two phases. The first phase used anaerobic and aerobic biologic treatment followed by clarification and filtration to clean fracture flowback and produced water at sufficient rates to support one or more fracturing crews on a continuous basis (Fig. 1).
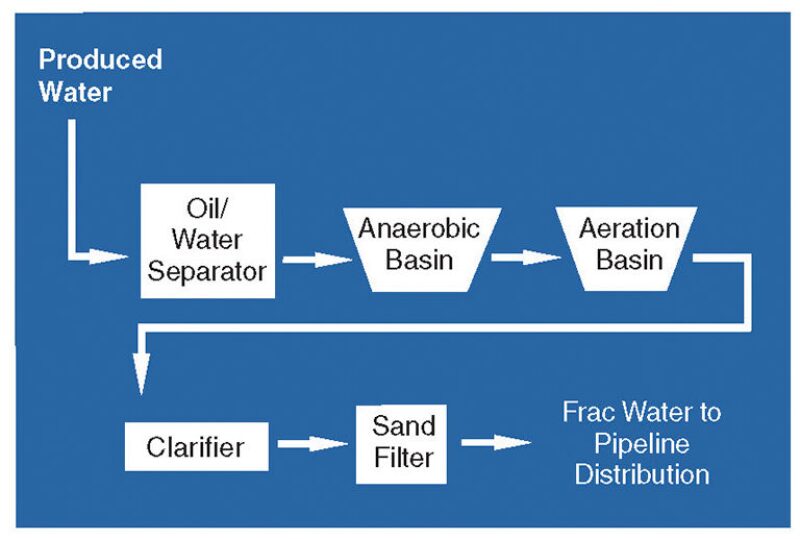
After the water has been filtered, it goes to a surge tank, which feeds the high-pressure pumps on the fracture water pipeline. The water redistribution line was constructed from idle gas gathering lines in the field. The shipping pumps are multistage centrifugal pumps installed with variable frequency drive-controlled motors. Using this configuration, the plant operators are able to rapidly adjust the shipping rate to match rate requests from the fracturing crews in the field. Taps are installed along the pipeline for connection through portable pressure reduction valve manifolds to high-density polyethylene (HDPE) lines strung along the roads to reach the fracture water tanks at the completion sites.
In the first four years of operation, the process cleaned and recycled 22 million bbl of fracture water. Water quality parameters for recycle water are shown in Table 2. Direct shipment to the fracture sites through the pipeline system is estimated to have saved more than 186,000 truck trips.
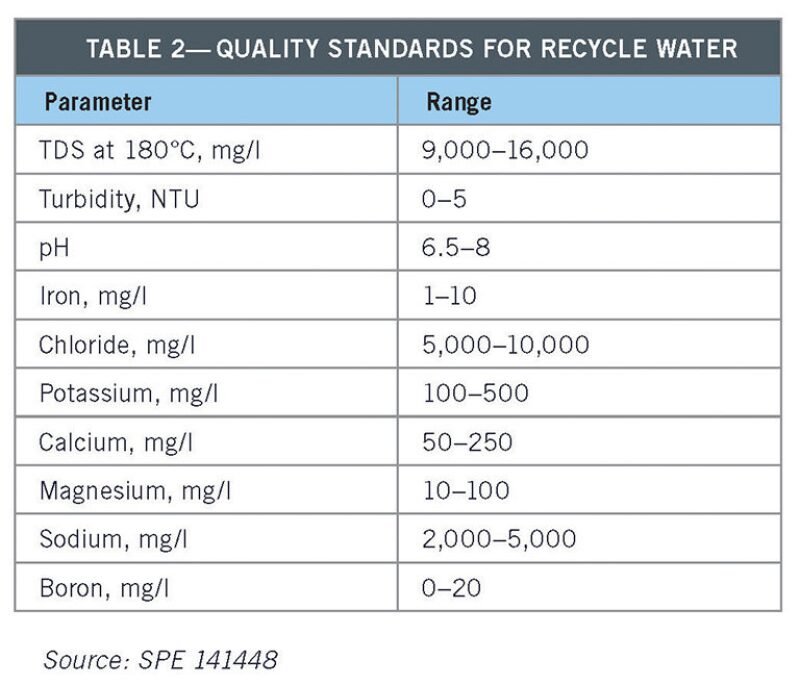
The second phase was designed to process surplus water to supplement freshwater supplies for drilling and cementing, surface pipe, surface construction, and other field development needs (Fig. 2). To meet discharge standards for a local river, constituents such as methanol, dissolved aromatic compounds, excess dissolved solids, and boron are removed using a membrane bioreactor, reverse osmosis, and ion exchange processes. Inorganic salts are reduced from more than 8,000 mg/l in the feed to less than 100 mg/l in the product water. Boron is reduced from levels of 15 mg/l to 30 mg/l in the feed to less than 0.75 mg/l, the drinking water standard, in the product water. Organic constituents are removed to below detection limits.
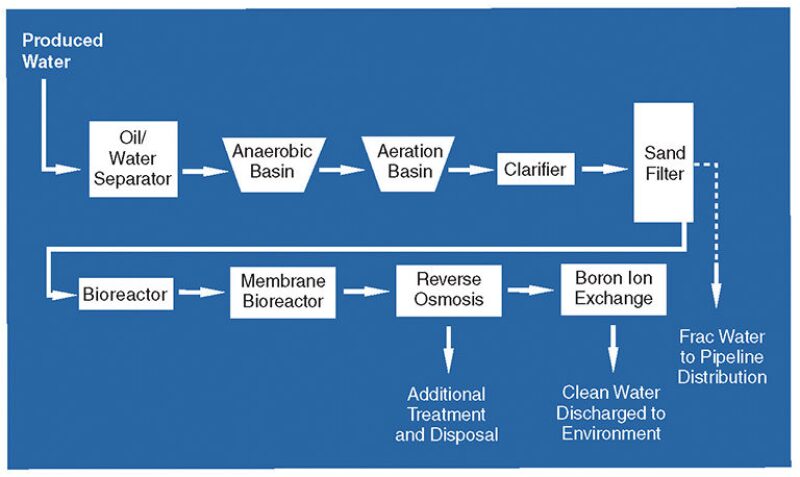
More than 200,000 bbl of processed water were used locally in the first year of operation with more than 1 million bbl of surplus water discharged to the river.
“The recycling of water is going to be primarily a logistics business with the main challenges being the economics, driven by transportation and storage. Treating the water for recycling is not that complicated,” Shafer said.
Granite Wash Water-Handling Infrastructure
LINN Energy completed the construction of and began using its water-handling infrastructure in the Granite Wash approximately a year ago. Covering a 16- to 18-sq-mile surface area in the Frye Ranch area in Wheeler County, Texas, the company uses a gathering system made up of a buried 8-in. HDPE pipeline to flow produced and flowback water from horizontal wells to a centrally located treatment facility for recycling and reuse.
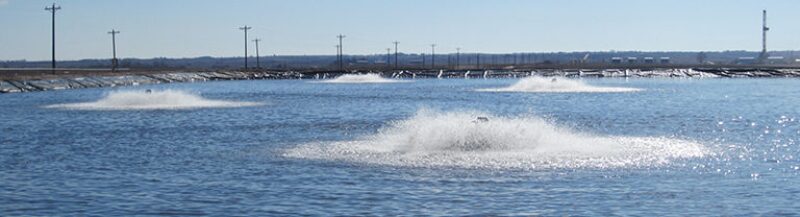
When the flowback water is delivered to the treatment facility, facultative aerobic bacteria are introduced into the tank battery gun barrel to digest any nutrients in the water that are favorable to anaerobic sulfate-reducing bacteria (SRB), which occur naturally in surface water. The digestion of the nutrients results in a reduction in the SRB population and minimizes the conversion of sulfate or sulfite to hydrogen sulfide, a byproduct which can lead to scaling.
The water is then run through a series of water tanks to remove as much of the remaining oil as possible; it is then discharged into a holding pond. More time is allowed for the aerobic bacteria to multiply and expend all of the available SRB nutrients. The company recently added four surface aerators in the holding pond to introduce additional oxygen into the water to further aid in the control of SRB.
The water then overflows into a second holding pond, ready for recycling as fracture water. Water samples taken from both holding ponds are tested for bacterial levels and water quality parameters. If the analysis results are within acceptable limits, the treated water from the second holding pond is then pumped back to wellsites for use as fracture water in well completions using a buried 16-in. HDPE pipeline and 12- and 10-in. HDPE lateral pipelines.
According to Dan Schilling, advisor engineer for water management at LINN Operating, although the front-end cost for the water-handling infrastructure is significant, the flexibility gained in the transport and recycling of water is cost-effective. “The economics are working for us given what we’re doing right now. We’re recycling water at considerably less cost than fracturing with only fresh water,” he said.
“Other operators are fraccing with fresh water only, buying water from water wells, surface ponds, wherever they can get it. They bear the cost of moving that water to a frac pit where it is treated with biocides and readied for the frac job. Once the water is used and brought back as flowback, they then also have to pay disposal costs. One of the offset operators has indicated that its disposal costs have been as high as USD 3.20 per bbl.”
Before the installation of the pipeline system, water was transported primarily by truck, and some was done with rental surface pipeline because of the large volumes of water involved. Schilling noted, “Some of these frac jobs are in excess of 200,000 bbl of water per well, with 10 to 12 stages per well.” This system is best suited to fields with multiwell drilling pads. The Granite Wash area leased by LINN Energy has multiple pay horizons with between three and six producing wells on each drilling pad, allowing for aggregation of produced and flowback water. After treatment, the recycled water is more efficiently returned to completion sites for successive fracturing of multiple wells.
The company is now developing similar systems in two other fields located about five to seven miles from the central treatment facility. The company plans to connect all three areas (including the existing Frye Ranch system) with a buried 16-in. HDPE water pipeline.
Mobile Water Treatment Technologies
Another alternative being used in unconventional resource fields are mobile water treatment technologies offered by various oilfield services companies, such as Halliburton, Baker Hughes, and M-I SWACO.
Clay Terry, strategic business manager of water solutions at Halliburton, highlighted the producers’ interests in water conservation. He cited the economic advantages to producers of reusing treated produced and flowback water, reducing their need for fresh water purchase, and the reduction of environmental impacts related to truck transportation.
Depending on the oil and gas development area, Terry said, mobile water treatment may be beneficial even in cases in which fixed facilities exist. When reducing reliance on truck hauling is desired or where there may be seasonal inaccessibility to wellsites or fixed facilities, mobile treatment alternatives may offer temporary options or may serve to augment the service area.
In assessment drilling programs in which operators have not yet made a commitment to full-scale development, the well count at a given time might be low, and the volumes of produced water will be small in comparison to a growing development scenario, noted Terry. Early in the development program, the operator will also have fewer options available to secure water resources or to reuse fluid volumes already paid for. As well construction activity increases, mobile treatment provides scalability to accommodate changing volumes of water.
Halliburton’s mobile CleanWave water service treats produced and flowback water to standards suitable for reuse in fracturing or drilling fluids. Using an electrical process, contaminated water is passed through the system’s electrocoagulation cells where the anodic process releases positively charged ions, which bind onto the negatively charged colloidal particles suspended in water. This results in coagulation. Gas bubbles produced at the cathode attach to the coagulated matter, causing it to float to the surface where it is removed by a surface skimmer. Heavier coagulants sink to the bottom, leaving clear water suitable for use in drilling and well construction operations.
Halliburton plans to expand its service into international unconventional plays this year, continuing its strategy of augmenting water supplies for well construction operations.
For Further Reading
SPE 119898 “Marcellus Shale Water Manangement Challenges in Pennsylvania” by A.W. Gaudlip, Range Resources Appalachia LLC, et al.
SPE 119478 “Frac Fluid Recycling and Water Conservation: A Case History” by D.V.S. Gupta and B.T. Hlidek, BJ Services
SPE 121104 “Breakthrough Mobile Water Treatment Converts 75% of Fracturing Flowback Fluid to Fresh Water and Lowers CO2 Emissions” by Aaron D. Horn, Newfield Exploration Mid-Continent
SPE 125336 “Optimizing Fracturing Fluids from Flowback Water” by S.M. Rimassa, Schlumberger, et al.
SPE 141448 “Water Recycling and Purification in the Pinedale Anticline Field: Results from the Anticline Disposal Project” by L. Shafer, Integrity Production Services