The success of water-conformance operations often depends on clear identification of the water-production mechanism. Such assessment can be complicated significantly when formation damage is also occurring. Coiled tubing (CT) and distributed temperature sensing (DTS) were combined to overcome challenging conditions (high temperature, low injectivity, high deviation, long perforated intervals, and wellbore damage) to identify damaged oil zones and suspected water-bearing zones in an onshore well in Japan.
Introduction
Source and location affect the selection of water-shutoff techniques, which are either mechanical or chemical in nature or a combination. No matter the operational approach, identification of the water zone is a critical step for any subsequent course of actions.
One method for the identification of water-producing zones is the use of production logging.
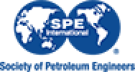