This paper describes challenges faced in a company’s first deepwater asset in Malaysia and the methods used to overcome these issues in the planning stage. Because of the depleted nature of all three reservoirs located above the untapped reservoir, a need exists to drill through these depleted intervals with an extremely narrow margin window (less than 0.5 lbm/gal). An added complexity is the location of the reservoir, directly below a hydrates bulge. To address the challenges of this well, an integrated approach between various disciplines has proved to be a critical success factor.
Introduction
The field is offshore Sabah. The first phase of the development was completed in 2014, with 16 wells drilled.
The development includes gas reinjection and seawater injection.
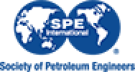