Tight gas reservoirs and shale gas reservoirs are economically viable hydrocarbon prospects that have proved to be successful in North America. In such reservoirs, established methods of well testing and data analysis are often impractical. This paper presents an integrated well-test program developed for a tight gas reservoir in southwestern China. The program, designed and modified from conventional methods to meet the project-delivery timeline and cost constraints, makes use of a combination of various formation-evaluation techniques.
Introduction
The northwestern and central areas of the Sichuan basin have been identified as having basin-centered gas potential. In particular, there are several blocky sand intervals in the Late Triassic Xujiahe formation that contain a relatively immature, continuous gas accumulation. The tight-gas-reservoir project described in this paper is located in the central uplift area of this basin and is operated by Shell and PetroChina as a joint venture. In this tight gas block, the Xujiahe formation has a gross thickness of greater than 500 m and is found at 3000- to 4000-m drilling depth. The hydrocarbon system in the block is an overpressured section of tight gas sands that are sourced by imbedded and surrounding shales and coals. Gas and condensate can be produced from this objective section through massive multistage hydraulic-fracturing treatments.
As with the exploration and development of any tight gas reservoir, the economic delivery of this project requires the integration of various well-testing methodologies to identify the economic feasibility of the reservoir. Well tests are normally conducted during various stages of the well life (Fig. 1). The objectives of well tests include the following:
- Identifying the hydrocarbon-bearing horizon
- Characterizing reservoir parameters
- Obtaining initial reservoir pressure
- Characterizing well damage and the extent of the damage
- Determining well deliverability and drainage area
- Evaluating well-completion efficiency
- Evaluating reservoir heterogeneities
- Estimating recoverable reserves

Unlike conventional hydrocarbon prospects, however, the formation permeability in a tight gas reservoir is extremely low, and commercial production generally requires stimulation through extensive hydraulic fracturing. The mature and well-defined methods of well testing and data analysis, although applicable, are often impractical because of excessive test duration. Therefore, the true potential in tight gas reservoirs can be established only when well tests are combined with a well-hydraulic-fracturing program.
We present an integrated well-test program developed and implemented for reservoir characterization and formation evaluation of this particular tight gas reservoir. The program makes use of a combination of various formation-evaluation techniques that include diagnostic fracture-injection tests (DFITs), perforation-inflow tests (PITs), pressure-buildup (PBU) tests, rate-transient analysis, and production logging.
Major Components of the Integrated Well-Test Program
DFIT. The DFIT is also sometimes called the minifracture test. Minifracture pumping is a routine part of a hydraulic-fracturing operation. DFITs provide information for fracture design (leakoff types) and reservoir properties (initial reservoir pressure and system permeability), which is used for predicting future production.
PIT. A PIT is a cost-effective technique for the evaluation of prefracture reservoir parameters. The PIT provides estimates of the reservoir pressure, flow capacity, gas-in-flow rate, and skin.
PBU. A PBU is the most familiar transient well-testing technique; it has been used extensively in the industry. The primary purpose of a PBU test is to estimate system permeability, initial reservoir pressure, and fracture half-length, height, and conductivity. In most tight gas reservoirs, however, the PBU test requires very long buildup times—months are typical—and supercharging. Therefore, the PBU test is often not feasible in very tight rock.
Production-Data Analysis (PDA). PDA includes a series of well-test techniques such as absolute open-flow potential, decline-curve analysis, rate-transient analysis, and the production-logging test. The purpose of these well-test techniques is to determine the well deliverability (including initial well potential), well-decline behavior for expected ultimate recovery, well drainage area, reservoir properties, and well-flow profiling.
Development of an Integrated Well-Test Program
The development of a tight gas reservoir generally requires extensive multistage hydraulic-fracturing jobs. Therefore, the integration of various well tests with multistage hydraulic-fracturing jobs becomes very complex, and a successful integration requires careful planning. To facilitate this integration process, we developed a decision flow chart. For a discussion of this flow chart and its application, please see the complete paper.
The ultimate selection of a well-test program depends on the balance between the cost of the program and the amount of data potentially acquired.
Well-Test Results, Challenges, and Solutions
Significant amounts of well-test data have been collected during the appraisal phase of this particular program. However, because of the limitation on the amount of data that can be practically presented in a single paper, we focus on the estimation of initial reservoir pressure. Initial reservoir pressure is one of the most critical reservoir parameters for estimation of the gas in place and recoverable reserves. For the purposes of comparison, we present the pressure-estimation results for the same fracture stage of a particular well by use of a PIT, a DFIT, and a PBU test.
PIT Results. A PIT was conducted in this particular well in the Xu4 formation at a depth of 3262 m. The well was perforated and allowed to bleed off for a short time period and then was shut in; this was followed quickly with a relatively higher rate (approximately 1,800 B/D) for approximately 0.32 hours. The well was then shut in for approximately 2 days, and the surface-pressure responses were recorded. An impulse plot yields an extrapolated initial reservoir pressure of 7,375 psi.
In summary, we found that PITs are successful when reservoir permeability is relatively high and good communication with far-field reservoir formations is established. However, PITs are unsuccessful when matrix permeability is low. Costwise, PITs are the most favorable option.
DFIT Results. A 1-day DFIT was executed successfully in the same formation of this particular well immediately following the PIT. During this test, a total of 88 bbl of water was injected into the formation, and the surface pressure was monitored for 16 hours. The DFIT data were analyzed by use of the standard software package. The recorded breakdown pressure at the surface was 8,214 psi, with an injection rate of 2 bbl/min. The maximum injection rate reached 10 bbl/min. The recorded surface maximum pressure reached 9,000 psi. The instantaneous shut-in pressure was estimated at 7,650 psi, corresponding to a bottomhole pressure of 12,286 psi. The after-closure analysis was used to estimate an initial reservoir pressure. The estimated initial reservoir pressure in this case was approximately 7,819 psi. This number is slightly higher than the 7,375 psi estimated from the PIT. The difference, however, is reasonable and within 6%. Also, the flow in this case is still in the pseudolinear-flow regime, and the pseudoradial flow has not yet started. Therefore, the initial reservoir pressure estimated from this test may contain some uncertainty, and that could contribute to the observed difference.
We observed that DFITs are successful when the formation can be broken down at the initial attempt. The success rate is approximately 70%, although operational cost is an issue.
PBU-Test Results. This particular well was put under production for a short time following completion of well flowback to clear up the completion fluid. The well was subsequently shut in for a total of 70 days while waiting for tie-in to the production pipeline. The tubinghead flow pressure and temperature were monitored during this time period (Fig. 2). The data were not used for pressure-transient analysis because of the presence of multiphase fluid inside the wellbore, and the depth of fluid contact was also unknown during this time period. A pressure and fluid-density survey was conducted with a production-logging tool at the end of the shut-in period. The results indicate that the reservoir pressure at the sand surface at a depth of 3262 m was 7,234 psi. This number is lower than those for the DFIT and the PIT. However, this is probably to be expected because the wellbore pressure had not yet completely reached equilibrium with the formation. Therefore, the pressure estimation from the pressure survey is generally consistent with the results from the PIT and DFIT.
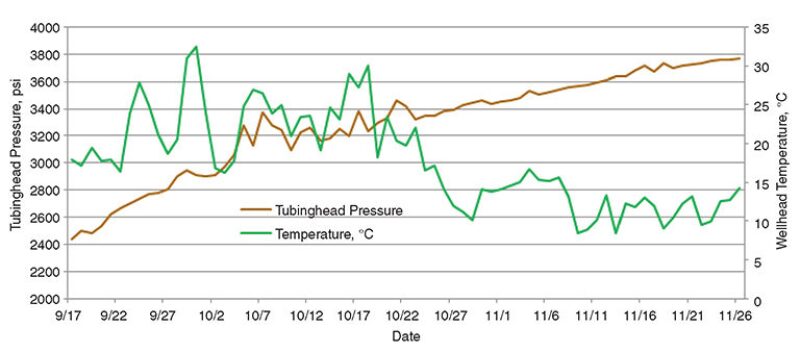
We found that PBU tests are the most expensive and time-consuming option and should be considered only when the well is idle, and should be coupled with a wellbore-fluid survey.
Challenges and Solutions
Not all of the well tests conducted in the field were successful. There were many challenges and issues during the conduct of the well-test program. For example, the initialization of breakdown of the reservoir formation becomes a major issue during the DFIT. In one such example, the formation failed to break down during several continuous attempts even though the bottomhole pressure reached 18,000 psi (approximately 6,000 psi above overburden pressure). Over the course of field operations, several actions were taken to mitigate this issue, including increasing the number of perforation shots and using better perforating tools, longer slugs, and acid and crosslinked gel. These and other actions did improve the success rate of subsequent DFITs.
This article, written by JPT Technology Editor Chris Carpenter, contains highlights of paper IPTC 16950, “Integrated Well-Test Strategy in Unconventional Tight Gas Reservoirs: Learning and Experiences From an Actual Field Project,” by Minquan Jin, SPE, Shell Exploration & Production; Wenxia Zhang, SPE, Shell China Exploration and Production; and Hongli Zhang, PetroChina Southwest, prepared for the 2013 International Petroleum Technology Conference, Beijing, 26–28 March. The paper has not been peer reviewed. Copyright 2013 International Petroleum Technology Conference. Reproduced by permission.