The complete paper presents a set of two- and three-phase slug-flow experiments conducted in a 766-m-long, 8-in. pipe at 45-bara pressure. The results show that the mean slug length initially increases with the distance from the inlet but that this increase slows and the mean slug length typically reaches a value between 20 and 50 diameters at the outlet. At low flow rates, slug-length distributions tend to be extremely wide.
×
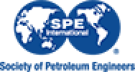
Continue Reading with SPE Membership
SPE Members: Please sign in at the top of the page for access to this member-exclusive content. If you are not a member and you find JPT content valuable, we encourage you to become a part of the SPE member community to gain full access.