Artificial lift is a critical technology used to keep wells producing when they are incapable of providing enough energy—in the form of pressure—to produce liquids to surface at economic rates. Most development plays throughout the world would be uneconomic without artificial lift. JPT Features Editor Joel Parshall, in his March 2013 JPT article titled, “Challenges, Opportunities Abound for Artificial Lift,” writes
There is no global repository of artificial lift statistics; however, industry observers estimate that 90% to 95% of the world’s producing wells currently use artificial lift, said Bill Lane, vice president of artificial lift systems emerging technologies at Weatherford. “It is trending more toward 95% than 90%, and probably 100% of producing wells would use artificial lift at some point in their lives, except for wells shut in prematurely because of economic factors.”
The 2014 SPE Artificial Lift Conference and Exhibition for North America, held in Houston 6–8 October, features a special Legends in Artificial Lift Luncheon on its final day. At the luncheon, five people who have dedicated their careers to artificial lift (AL) will be honored for their outstanding contributions to the field of AL technology. The SPE Legends of Artificial Lift Award recipients are Herald Warren Winkler, James F. Lea Jr., Maurice Patterson, Sam Gavin Gibbs, and Joe Dunn Clegg. Serving on the SPE Board as 2014 SPE Technical Director for Production and Operations, I am proud to host the ceremony along with 2014 SPE President Jeff Spath, who will give an address and present the special recognition awards to the five men whose lifework distinguishes them as AL legends.
Each honoree is being recognized for accomplishments in a specific type of artificial lift—or as a champion of all AL techniques. These individuals’ curiosity, keen observation, and dedication to physical truths have, time and time again, trumped conventional wisdom, revealed ineffective techniques and exaggerated product claims, and led to forward-looking insights that have until this day driven many AL advancements in understanding, technology, and techniques. For many production engineers—including me—these five modest men are influential mentors whose work has played a major role in making the AL industry such an exciting and rewarding field to work in.
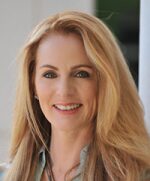
Shauna Noonan, SPE, is completion technology manager for ConocoPhillips in Houston. She has worked on artificial lift and technology development projects worldwide at ConocoPhillips and previously at Chevron for 20 years. Noonan has chaired many industry artificial lift forums and has authored or coauthored numerous papers on the subject of artificial lift. She holds a position on the SPE International Board of Directors as the 2013–15 Technical Director for Production and Operations and serves on the JPT Editorial Committee. Noonan also received the 2012 SPE Gulf Coast Section Regional Production and Operations Award. She began her career with Chevron Canada Resources and holds a BS degree in petroleum engineering from the University of Alberta.
Meet the Legends
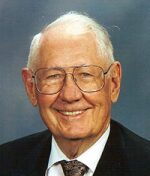
Joe Dunn Clegg
“When I was growing up, my father worked for the Texas Company in various fields throughout Texas,” said Joe Clegg. “This was during the Great Depression, and he had a good-paying job. We were very fortunate.”
Clegg’s older brother graduated from Rice University in 1943, served in the US Army during World War II, and went to work for Exxon when he came home.
In June 1945 when he was 17, Clegg was drafted into the US Army. “By the time the war was over,” he said, “I’d finished basic training. I did go to Germany, but I was in the occupation forces.”
Upon returning, it was natural for him to study mechanical engineering at Rice. After earning his 5-year bachelor’s degree, Clegg was recruited by Shell. He spent his first year in training. “You had to do roustabouting and roughnecking,” he explained. “You worked with the lease operator and then with the various types of engineers, a week with each. We also got to go to mud school—not med school, but mud school—where you learned all about mud.”
Then, in the Permian Basin area around Midland, Texas, Clegg worked for 7 years in the field as a mechanical engineer.
“In 1952, Shell had two types of engineers—exploitation engineers and mechanical engineers,” said Clegg. “The mechanicals, we designed the casing and the cement job. We put the pumping units on the wells. That’s where I first became involved with artificial lift. We also built the tank batteries. So our business was to get the oil from the wells to the pipelines. Exploitation engineers were concerned with problems in the reservoirs.”
In 1960, he was assigned to the Midland area office, specializing in surveillance and artificial lift.
“Then as now, by far the biggest type of artificial lift in west Texas was sucker-rod pumping,” said Clegg. “There were a few gas-lift wells. Most of the stuff out there was low volume, so those tended to be intermittent gas lift. There were a few hydraulics, a few electrical submersible pumps. Essentially no progressing cavity pumps. Plunger lift—very limited application back then.”
Clegg started off designing beam pumping units for fairly shallow wells. “As you got more experienced, you got the more complex, deeper wells,” he said. “I ended up with fairly deep, high-pressure gas-lift wells and some deep, complex dual gas-lift wells.”
Selecting, designing, and then making sure the unit was installed correctly involved attending to many details.
“You don’t have to be there to see them pour the unit foundation,” explained Clegg, “but maybe you ought to review the design and decide whether you want a permanent concrete base or to buy bases you can move around. You need to be familiar with what’s done. For instance, the polished rod that you’re going to put on at the top needs to be perfectly straight up and down so that you don’t wear out the stuffing box and the top portion of tubing. Little things like that I’d quickly recognize and the people in the field should know about too. But sometimes they’re missed.”
On occasion, someone would forget to fill up the gearbox. “They don’t run very long if they don’t have oil in them,” he said.
Writing up the specifications was very important. “You selected three reliable manufacturers to get bids from,” Clegg said. “There’s a lot of little things. What kind of prime mover are you going to use? If you want an electric prime mover, do you need it elevated or sitting on a flat base? If water tends to rise at the location, you’d need high prime—setting the motor maybe 2 feet off the ground.”
Clegg has been at only one location where Shell generated its own electricity. “It was a platform off Los Angeles—Long Beach. We did that in about 1985,” he said. “It has electrical submersible pumps. It’s been operating for a lot of years. Haven’t heard about any problems.”
Basic spec choices make a big difference. “With beam pumping, at 10 strokes a minute, you need a certain size sheave, with four or five belts on it,” explained Clegg. “You don’t want the belts too big because they won’t bend around the sheave as easily. You don’t want them too little because then you’ll need too many belts. You have to have some sort of awareness of all that. So with the sheave, you have a smaller one on the motor—maybe 10 inches—where the one on the unit may be 30 inches—it’s a great big one. Then the pumping unit will run at 10 strokes a minute.”
Manufacturers’ catalogs list the formulas needed to calculate what combination of equipment is needed to achieve a correct pumping rate for the well. “Typical beam pumping units, you run at 10 strokes per minute,” said Clegg. “Most units, you seldom run them more than 20 strokes per minute. That’s going pretty fast. You have to know the gear ratio, beam diameter, speed of the motor.”
During 1983 and 1984, Clegg worked in hydraulic fracture design programs. The following year, he was switched to completing wells in the Atlantic Ocean off the coast of New Jersey. “We were drilling in 7,000 feet of water,” he said. “My boss said, ‘If we make the evening news, you’re fired!’ Well, we didn’t even find any oil to produce.”
By this time, Clegg was a recognized expert in all types of artificial lift. He was chosen to serve during 1984–85 as an SPE Distinguished Lecturer. His topic was “Artificial Lift: Producing at Optimal Rates.” In 1988, SPE presented him with its Production and Operations Award. For outstanding achievements in artificial lift, Clegg received the J.C. Slonneger Award in 1989 from the Southwest Petroleum Short Course. During 1993–94 he served a second time as a Distinguished Lecturer, speaking on “High-Volume Artificial Lift.” Clegg received the Artificial Lift R&D Council’s 2013 Artificial Lift Award.
After retiring from Shell in 1991, he began teaching for Oil & Gas Consultants International. Clegg was a natural. He taught the Production Operations course over the next 10 years—in the US, Canada, England, Scotland, Austria, Chile, the Netherlands, Denmark, Angola, Indonesia, India, and Pakistan.
Clegg has also worked on consulting projects and been active in the American Petroleum Institute (API) as a member of the Gas Lift Equipment Task Group, as well as the original API committee, 1972 to 1974, that wrote standards 14A and 14B on subsurface safety valves. He has authored and presented several papers and served for many years on SPE’s annual conference program committee as well as the JPT Editorial Committee.
Around 1999, Clegg agreed to serve as the editor for the fourth volume of SPE’s Production Engineering Handbook, published in 2007–16 chapters covering all the major aspects of production operations engineering. Clegg coordinated and oversaw the entire 909-page volume over the course of 6 years—working with each subject expert, editing drafts, ensuring all chapters were within page-number limits.
“We looked at that book as our bible,” said Shauna Noonan, ConocoPhillips. “You can rely on it. It’s really all the essentials you need to know about artificial lift.”
And about production operations. Clegg’s chapter, which he wrote with Erich Klementich, was on tubing selection, design, and installation. He knows about that, too.
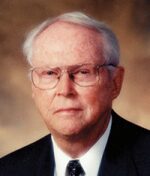
Sam Gavin Gibbs
When Sam Gibbs was drafted into the US Army in October 1954, he was sent to Alaska as a computer operator in a 120-mm gun battery defending Eielson Air Force Base. “I was awed by the aurora borealis and the long star-filled night skies in winter,” he said. “I became interested in astronomy and celestial mechanics. I set out to calculate an ephemeris of Earth positions as it orbited around the sun.”
Although he had just graduated from Texas A&M University and been hired by Shell as a mechanical engineer, Gibbs found he could not make the ephemeris calculations. “Realizing I was mathematically illiterate was a life-changing experience,” he said.
Returning in late 1956 to Shell’s training program, he worked as a roughneck on a steam drilling rig at Weeks Island, south Louisiana; in a roustabout gang in Texas; and in production accounting. He also took crash courses in petrophysics and geology. Following training, Shell assigned him to the Midland, Texas, area as unit engineer in Garza and Borden counties. He designed tank batteries, built low crossings, and made drilling authorizations for expenditure (AFEs). “That was where I learned that ‘transportation and contingencies’ was the number you added to the bottom of the AFE to make it come out even,” he said.
And he puzzled over what the mysterious dynamometer cards meant, initialed them, and threw them into his “Out” basket.
“Frankly, I hated it,” he said.
So he resigned from Shell and returned to Texas A&M. He had made friends among the professors. “They designed a curriculum for me,” Gibbs said, “to turn a frustrated engineer into a mathematician.”
What he learned gave him life-changing insights. “In the appendix of a differential equations book,” he said, “I found an article about British physicians who used Fourier analysis to interpret human electrocardiograms. I remembered those mysterious dynamometer cards in Midland.
“Then it came to me: The analogy between electrocardiograms and dynamometer cards is perfect! The downhole pump in the well is the human heart in electrocardiograms. Tiny electrical currents cause the heart to beat. These currents travel through bodily tissue to electrodes that have been placed noninvasively on the patient’s skin. The signals are recorded on strip charts and interpreted with the aid of Fourier analysis.
“In the oil well, valves open and close in the downhole pump, causing stress waves to travel to the surface through the rod string. The stress waves could be recorded at the surface with a dynamometer located at the top of the rod string. The signals from below could be interpreted with the wave equation of mathematical physics.”
The other ‘aha!’ moment involved the wave equation that arises from Newtonian dynamics and Hooke’s law of elasticity.
On a day he had intended to cut class and play golf, Gibbs learned from his professor Dr. Luther about a special property of the wave equation—that a solution to a partial differential equation problem could be obtained with the finite difference method. “So the size of the increments on the rod string didn’t matter,” said Gibbs. “This meant that an accurate design program for rod-pumping installations could be obtained with the slow computers of the day.”
He paused. “I still occasionally look at the dog-eared page in that book.”
After graduating with a master’s degree in mathematics, he accepted Shell’s offer to become a researcher in the Shell exploration and production laboratory in Houston.
“My boss’s boss—his name was Bob McEntee—he loved rod pumping,” said Gibbs.
McEntee assigned Gibbs to rod pumping research. The first thing Gibbs did was work on a mathematical model of the pumping system. Using the wave equation and its special property, within a few months he modeled the prime mover, the pumping unit, the rod string, and the downhole pump. “The method worked great,” he said. “Shell programmers prepared a computer program and soon the company began designing rod pumping installations with the wave equation.”
Gibbs then worked on what was to become the “Diagnostic Technique.”
“I developed a classical Fourier series type of solution like the British physicians had with electrocardiograms,” he explained.
But the state-of-the-art Johnson-Fagg mechanical dynamometer plotted surface load vs surface position, with no sense of time. So a new type of dynamometer was built by Shell’s instrumentation group, led by Bob Kolb.
It worked in the field. “The procedure was inefficient and uneconomical,” said Gibbs. “But it spoke the truth.”
Shell then sent Gibbs to Rice University to earn a PhD while working. His thesis was on irregular ocean waves. “I never found application for this as a west Texas well weigher,” he said.
After graduating, he soon was made the company’s division engineer at its New Orleans location where his friend Ken Nolen worked as production engineering section head. But Gibbs wanted to solve problems in the field, not the office or classroom. So he quit Shell and turned down an offer from the University of Texas to become a petroleum engineering professor.
By 1971, Gibbs and Nolen had formed Nabla Corporation and Shell granted them a license to use the Diagnostic Technique. “We put the Diagnostic Technique in a computer in a truck and hauled it to the well site and did computations right there so we could hand the customer the diagnosis with a completed report right at the well site,” said Gibbs.
He and Nolen carried their work to the field for the next 26 years. “The predictive wave equation work I’d done was never patented,” he explained. “We carried it much further. We taught people how to design deviated wells. We developed a pump-off controller algorithm we called the Pump Card Monitor. It was a big hit.”
After he and Nolen sold Nabla to Lufkin in 1997, Gibbs consulted at Lufkin for 2 years to help the company understand Nabla’s products.
“But the inventive juices still bubbled within us,” he said. He and Nolen developed the “Inferred Production” method for making continuous production tests using the downhole pump as a meter. And Nolen helped him generalize the pump card algorithm in the SAM Well Manager to handle deviated wells. “Friction is different in a crooked well,” explained Gibbs.
Gibbs has received many honors. He served as an SPE Distinguished Lecturer during 1988–89. The SPE Permian Basin Section elected him to their Hall of Fame in 1992. Gibbs received the Southwest Petroleum Short Course’s J.C. Slonneger Award (1987) and Duane A. Crawford Service Award (1996), as well as the SPE Production and Operations Award (2001). On 15 March 2011, the Texas House of Representatives passed a resolution “honoring Sam Gavin Gibbs of Midland for his induction into the Petroleum Hall of Fame” at the Petroleum Museum in Midland, Texas.
But what really makes Gibbs tick can be found in the “Closure” section at the end of chapter 3 in his book Rod Pumping: Modern Methods of Design, Diagnosis, and Surveillance, published in 2012. Echoing the words artificial lift pioneer Walton Gilbert imprinted on his memory in the late 1960s, Gibbs gives his readers complex, practical advice in two simple words, “Think downhole.”
Because that’s where the pump lives—the system’s heart.
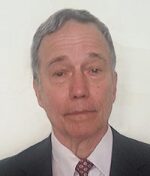
James F. Lea Jr.
“Why did I choose mechanical engineering?” said Jim Lea. “Probably thinking about a future in aerospace.”
After earning a bachelor’s degree in mechanical engineering in 1965 at University of Arkansas, Lea began his career working on rocket engines for Pratt & Whitney. “I was in a group that was developing smaller engines for the upper modules of rockets used to lift heavy payloads into orbit or even go to the moon,” he said.
But after a few months, Lea concluded that cutbacks were on the horizon for government-sponsored research. He returned to mechanical engineering studies at the University of Arkansas and earned an MS degree in 1967, then went to Southern Methodist University and received a PhD in 1970.
Although his next employer’s name was Sun, its focus was on Earth and the oil beneath its surface.
At Sun Oil in Richardson, Texas, Lea worked as a senior research engineer, focusing on fluid flow, thermal stimulation, and secondary recovery. “I also got involved in a project testing a hovercraft drilling barge,” he said, “to see if it could be used for drilling up in the high Arctic.”
He went up to the Great Slave Lake in Canada’s Northwest Territories. “It was the middle of winter and 50 below zero,” Lea said. “We pulled this thing back and forth across the lake. It was actually an ice breaker, as it turned out. It pushed the air underneath the ice, the ice would lose its support from the water, and the ice would break. I don’t think they ever used it for drilling. But they did wind up using transportation hovercrafts up and down the rivers in Canada maybe partially as a result of the test.”
Lea was issued eight patents (on Sun Oil’s behalf) on systems, methods, and apparatus related to his Arctic research. By 1975, however, Sun was experiencing some difficulties, so Lea decided to try a different approach to his career. “I thought that teaching would be kind of nice,” he said. “Working with students, not being under corporate guidelines…”
So Lea accepted a position at the University of Arkansas as an assistant professor and taught courses in fluid mechanics, statics and dynamics, strength of materials, and engineering analysis. While there, he was awarded a National Science Foundation (NSF) research contract. “I don’t know how I got it,” he said. “People can spend their whole careers trying to and never do. I would say it was the only NSF contract awarded to college engineering up to that time.” In a continuation of the interest he had developed at Sun, the NSF research was to study how engine waste heat could be used in the Arctic to heat the hull of a drilling barge, assisting in station-keeping atop ice-covered waters.
During the summers, he started working as a student at Amoco Production Research in Tulsa. In contrast, the realities of university life began to weigh on him. “It was not as rosy as I thought,” said Lea. He was offered a full-time position as a research associate at Amoco. He accepted.
It was a job that was about as close as Lea could get in the corporate world to what he sought in academia—“and it didn’t have the worrisome stuff,” he said. “It’s not that I didn’t have an argument or two with the boss, but he was actually a decent guy. I always respected him.”
In Amoco’s production-focused research department, Lea was in the midst of 600 other researchers. It was then that he started to work in artificial lift. “I’d always kind of looked at Kermit Brown, who wrote all these books on artificial lift and lived in Tulsa,” he explained. “He seemed to be enjoying life, getting along pretty well, focusing on lift. Winkler—I knew him because he used to come teach courses at Amoco. So I met him, talked with him. And I thought, ‘Well, he likes this artificial lift. Well, OK. And he thinks it’s great.’ So I got to working in that area somewhat due to their encouragement.”
He helped create new design and analysis computer programs for lifted and flowing wells. “We’d take these programs all over the world, install them, and they would use them,” said Lea. “Like one time I walked into the office in Egypt and looked across the room. And there are all these computer screens and on every one of them they had one of our programs. They were using them. I guess we’re not doing so bad here! It was kind of encouraging.”
Lea conducted tests at Amoco Research to study how much gas is tolerated by an electrical submersible pump before performance degradation. He ran a field experimental test program where beam pump gearbox torque was measured while the unit was running. He created a mathematical model of the intermittent gas lift method using plunger lift. The model tracks the plunger as a function of real time, relying on experimental expressions for liquid fallback.
“It was good at Amoco because they encouraged publication,” said Lea. “A lot of companies don’t do that. But there they used it as a way of showing the progress of research. They also showed publications to upper management to justify funding requests.”
During 1987–88, he served as an SPE Distinguished Lecturer, speaking on “Artificial Lift: Minimizing Operational Costs.” He was chosen again to serve as a Distinguished Lecturer, this time during 1994–95, lecturing on “Emerging Technologies in Artificial Lift.” In the late 1980s, Herald Winkler asked Lea to join him in writing a two-part series of articles for World Oil, titled “What’s New in Artificial Lift.” Little did either man know that this was to become, with each annual pair of articles over a more-than-25-year timespan, a valuable record of trends, fads, and genuine development in artificial lift.
In 1990, Lea received the J.C. Slonneger Award, for outstanding contributions to the field of artificial lift, from the Southwest Petroleum Short Course. SPE presented him with its Production and Operations Award in 1996.
Then, in 1999, BP merged with Amoco. “It was kind of a shock to me that Amoco disappeared,” he said. “I was as high up with Amoco as I wanted to be. I would have stayed there forever.”
Lea had the opportunity to chair the petroleum engineering department at Texas Tech University, where he served until 2005. There he started the Gas Well Dewatering Workshop—which has become an annual event, now managed by Cleon Dunham, drawing around 700 attendees—and coauthored a book titledComplete Gas Well Deliquification.
Now Lea is in another, unexpected and rewarding, phase of his career. He teaches five 1-week courses for PetroSkills: overall artificial lift, plunger lift, gas well deliquification, electrical submersible pumps, and beam pumping. He continues to be involved in complex consulting projects; organized a new consortium, Production and Lift Optimization; and works with Lufkin Industries, now part of GE.
“When I was in high school,” said Lea, “I won the state meet in the 100-yard dash.”
Although these days he walks and bicycles, his career continues, full speed ahead.
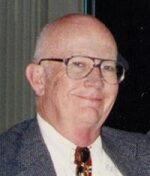
Maurice M. Patterson
In high school, Maurice Patterson’s best friend’s father was an engineer for Cameron Iron Works. “I was good at math and physics,” said Patterson. “I decided that maybe engineering was the place for me.” So he talked to his friend’s dad.
“When I got to Rice University,” he said, “I wanted to be a chemical engineer. I found out I had no aptitude for chemistry, so I went into mechanical engineering.”
After graduating in 1954 with a bachelor’s degree in mechanical engineering, Patterson went to work for Union Carbide. “It was a total disaster,” he said.
Patterson and his fellow trainees had to move 50-lb barrels of a dry catalyst from the ground and dump the contents into a tank. “We figured out how to do it without hauling,” he said. “We just pressurized the chemical powder and blew it up and into the tank. We took our invention in to the boss, we were so excited. The boss said, ‘Ppthww! Hauling, that’s what you’re here for.’ And he threw it into the wastepaper basket. I decided to quit right then.”
Patterson joined a small Houston firm called Anderson, Greenwood & Company, a valve designer and manufacturer acquired by Keystone Valve in 1986. “They made parts for missiles and aircraft,” he said. “In fact, we did the whole tail assembly for the KB47 tanker. We designed it and built a mockup.”
Following a stint in the US Army, he decided to earn a master’s degree in mechanical engineering at Rice. Upon graduating, he only wanted to interview with oil companies—to work in the field, not in refining. “I’m glad I went to work for Shell,” he said. “Unbelievable career.”
He started out in the company’s training program in 1960. “I spent from September until the next March between Glendive, Montana, and Minot, North Dakota,” said Patterson. “They were just developing the big anticline on the east. But it was cold—it was really cold up there—20 below zero.”
Next was “Geology School,” taught by Geology Department professors at Texas A&M University. “Four weeks of pure hell,” said Patterson. “Forty guys in a class. It was a great course. It opened your head and crammed 3 years of geology into it.”
After working with Shell’s physics department designing underwater equipment that “sniffed out” methane seeps for potential field development, he transferred to Shell Research’s Bellaire location. There he worked on the Oceanographic Data Gathering Program analyzing data gathered by specially designed instruments installed in 1968 on Gulf of Mexico deepwater platforms to measure and record storm data. On 17 August 1969, Hurricane Camille passed between two of the monitoring stations. “When we got the data back, it was on a big roll,” said Patterson. “Ocean engineer Gordon Sterling and I, we were at the New Orleans Roosevelt Hotel on the third floor, and we laid it all out from one end of the corridor to the other. We could really get a picture of it—50-, 60-, 75-foot waves. We proved that the period between the waves was nowhere like what people had predicted. It was a lot less.”
Then he discovered artificial lift. “A lot of the pump jacks were being overstressed because of too much torque,” said Patterson. “Division engineer Danny Johns said, ‘Can you find out why the gearboxes are failing?’”
“Strain gauges were pretty popular,” he explained. “But nobody knew how to measure torque with them.”
The strain gauge was tiny but extremely accurate. It was glued to the steel drive shaft, current run to it through wires, and the change in resistance was measured. Patterson and Johns spent a month gathering the data and another month analyzing it. “We found the 640 drive shaft, built to handle 640 in./lb of torque, was putting over 900,000 in./lb of torque,” Patterson said. “We were trying to get all the oil out of the ground—speeding them up, changing the stroke length, that sort of thing.”
“We worked with a guy from Lufkin [Industries] to get the gearboxes changed to handle that kind of torque,” he said. “I think they just changed the shape of the gear face and the pitch of the gear teeth.”
In the latter part of the 1970s, Patterson—along with colleagues Joe Clegg, Buford Neely, Danny Johns, and Cleon Dunham—started realizing that rod pumps could be monitored to figure out when they pumped off. This was the beginning of pump-off control. “Then we began to instrument the field,” he said. “We began to realize that by monitoring rod pumps, maintenance went down and production went up. So we did that and profits got a lot bigger.”
During that same period, Patterson also began electrical submersible pump (ESP) research and testing. Neely and Patterson developed a soft starter—a reduced-voltage starter that eliminated the detrimental effects of cycling. The starting current was reduced to half or less of normally expected starting current, and the time required to bring the motor up to full speed was increased by a factor of 5 or 6.
“ESP manufacturers were selling one thing that wasn’t working—the cable,” he said. “So in the 1980s, we tested all the cables we were supplied. We took a pressure vessel, about 10 feet long, and ran a cable all the way through it and out the bottom. Then we put one fluid in the casing inside the pressure vessel and turned the power up. There was no drain on it—just straight power—and we heated them up to about 250 degrees. Man! We blew cables out everywhere. The biggest mess you’ve ever seen. Couple of the cables made it. So that became the recommendation for the ESP.”
For the final 3 years of his career, Patterson worked in operations as senior staff, primarily to aid foremen in the transition to field operations computer monitoring and control. It could be a tough sell. “The computer was such a change to their way of life,” he said. “They were still spending their time driving around and looking at wells. I kept saying, it’s on the computer.” However, Patterson’s way of dealing with crusty foremen resistant to computer control drew their respect and often resulted in good friendships.
Patterson retired in 1989, working as a mechanical engineering consultant for the following 20 years. He now lives far from big-city life, in Hemphill, a small town in east Texas near the Louisiana border.
He has served as an SPE Distinguished Lecturer (1990–91), worked on many SPE and OTC committees, and participated often in the ESP Workshop. He has authored or coauthored several seminal papers and 14 patents. But he has not had a résumé since he was invited to join Shell in 1960. “I was so happy,” said Patterson. “I wasn’t about to go looking for another job.”
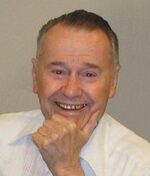
Herald Warren Winkler
Herald Winkler’s first job was in Denver City, Texas, working as a production engineer for Atlantic Refining Company. He had just graduated from Southern Methodist University with a BS degree in mechanical engineering. “When I entered the oil field in 1948,” Winkler writes in a brief personal history, “a barrel of oil from Saudi Arabia could be delivered to a Houston refinery for less than USD 2 per barrel and a company was lucky to find a buyer for natural gas at 5 cents per Mscf.”
As a production engineer, he was responsible for ensuring that producing wells maintained target oil and gas production rates. He became adept at well testing, solving gas/oil ratio control problems, and dealing with water coning and corrosion issues. His best teachers were field workers, artificial lift equipment operating in the field, and the actual well itself. “I got along great with the pumpers,” Winkler said. “If I had a problem, they’d save me. I ate with them and worked with them.”
Winkler gradually learned how to select artificial lift and surface equipment as well as maintain it in the field—attempting to prevent wear, corrosion, and overworking or underworking the system; reduce equipment failures; ensure production levels consistent with the company’s ultimate recovery estimates; and increase production by judiciously selecting wells for workover.
Starting in 1952, Winkler continued his experience-based learning as district manager at Camco Gas Lift Company. Over the next couple of years, his understanding of artificial lift—particularly gas lift—grew as he maintained a gas-lift equipment inventory and repair shop, designed gas-lift systems, installed gas-lift valves for lifting oil wells and dewatering gas wells, and worked on early oilfield automation systems.
When he began a 16-year stint in 1954 as Camco Inc.’s gas-lift technology and products manager, Winkler’s development intensified. The way he puts it, “I was a troubleshooter. When a customer was ready to kill the next Camco person they saw, that’s when they called me in.”
Traveling worldwide, Winkler designed and installed gas-lift systems; supervised field operations; and analyzed, troubleshot, and researched most phases of gas-lift operations. He built the first gas-lift valve stem travel probe and a test facility for dynamic performance testing of all widely marketed gas-lift valves. He presented gas-lift production courses and seminars in most oil-producing countries in the world.
During those years, he also earned degrees in petroleum engineering, working 6 years at night to earn an MS degree in 1962 at University of Houston, then another 4½ years to earn a PhD in 1969 at The University of Texas at Austin.
And while he studied and worked, others beyond Camco also benefited. “Every time I learned something at work, or learned you actually could do something when someone said you couldn’t,” said Winkler, “I wrote about it so others wouldn’t screw up like I or someone else did. It had nothing to do with building up my name.”
Starting in 1954 with the second annual West Texas Short Course on Oil Lifting [now a nonprofit education organization called the Southwestern Petroleum Short Course Association (SWPSC) headquartered in the Texas Tech University Petroleum Engineering Building], he began this practice of distilling what he had learned into the written word—resulting in well over 130 practical papers, articles, presentations, manuals, and book chapters. For example, he wrote a seven-part series of articles for World Oil titled “Here’s How to Improve Your Gas Lift Installations”—one a month from August 1959 to February 1960. The series basically constitutes a practical course readers can use on the job, presenting a simplified method for constructing gas pressure at depth curves, a simplified valve spacing chart for intermittent gas-lift design, a delineation of injection gas requirements for intermittent lift, and a description of how one estimates injection gas volumes used by intermittent-lift wells.
The analysis and insight Winkler articulated in his writing, courses, and on-the-job problem-solving led to his appointment in 1970 as a professor in the Department of Petroleum Engineering at Texas Tech in Lubbock, Texas. He served as department chair from 1971 to 1976. Winkler taught most methods of artificial lift, including sucker-rod and electrical submersible pumping, hydraulic jet pumping, and gas-lift systems. He also taught multiphase flow theory and application of gradient curves; flowing oil and gas well analysis; and engineering analysis using FORTRAN programming, directional drilling calculations, and casing design. During the summers, he worked as a consultant, presenting workshops and seminars as well as analyzing, evaluating, and reviewing gas-lift operations at international and national oil companies all over the world.
From 1985 to this day, Winkler has served Texas Tech as professor emeritus of petroleum engineering.
Winkler has received many honors: SPE’s Lester C. Uren Award (1976), SPE Distinguished Membership, the Production Award from the Petroleum Division of the American Society of Mechanical Engineers (1983), SWPSC’s J.C. Slonneger Award (1984), and the Artificial Lift Research and Development Council’s first annual Award for Distinguished Service to the Artificial Lift Industry (2009).
Such things, however, are not what drives him. “The two most important decisions in my life,” he wrote, “were choosing my partner and my occupation.”
During the 1960s, when a competitor offered Winkler twice the salary he was making at Camco, he turned the offer down. He could do the kind of work he wanted to at Camco—and in the uncompromising way that suited him. “My manager told me, ‘If you were a little more political, you’d be Camco’s president,’” said Winkler. “But I did tell him the truth. He could always count on me.”
Winkler’s 90th birthday was 18 August this year. His beloved wife Anne—his partner for 64 years—was not at his side. She died in 2008. “If I was God,” said Winkler, “I can’t think of a thing I would change in her. She was perfect. Do you know what the last thing she said to me was? ‘I love you.’”