This paper presents a case history of a North Sea well in which managed-pressure drilling (MPD) was used as the enabling technology to drill, case, and cement the well, with particular focus on the planning process, design methodology, and execution particulars. A novel fluid-pressure-transmission pill (FPTP), composed of a crosslinked polymer, was also used to maintain hydrostatic pressure while tripping bottomhole assemblies (BHAs) and during deployment of the liner and sand-control equipment.
Background and Drilling Challenges
The Harding field is a mature North Sea platform development upon which an infill-drilling project has been initiated to access the remaining oil. This oil tends to lie in more-distant, hard-to-reach targets, including those in the far north of the field and a new field located south of the platform. These would be accessed by extended-reach-drilling (ERD) wells. A combination of reservoir depletion and weak interbedded sands and shales has resulted in a further reduction in the already narrow mud-weight (MW) window between fracture and formation-collapse pressures. The window had decreased from 2 to 0.7 lbm/gal, equivalent to a 200‑psi bottomhole-pressure (BHP) window. ERD to access the oil accumulations most distant from the platform would generate equivalent circulating densities that are 50% greater than the available MW window, so these wells are not conventionally drillable.
After MPD proved to be an effective strategy for a test well (IC6) in the Harding field, the decision was made to apply MPD to two ERD wells (PNE4 and PNE2) that had not achieved their objectives. A subsequent investigation into the shortcomings of these wells led by the subsurface team resulted in a reworking of the pore-pressure/fracture-gradient predictions for the northeast of the field, taking the depletion in that part of the reservoir into account. Sand/shale interfaces at the margins of the reservoir, and sand/sand interfaces created by the injection of sand through the main reservoir body, also appear to result in localized fracture-gradient reduction. These combined factors resulted in a reduced MW window compared with that previously used in well planning. Hydraulics modeling strongly suggested that drilling PNE2a (the redrill of PNE2) would be unachievable conventionally, and drove the decision to use MPD for the reattempt. PNE2a would include a 12¼-in. overburden section, a highly challenging prospect containing more than 6,000 ft of shale, as well as the 8½-in. reservoir section, which was expected to have significant sections of shale where instability had previously caused drilling problems (see Fig. 1).
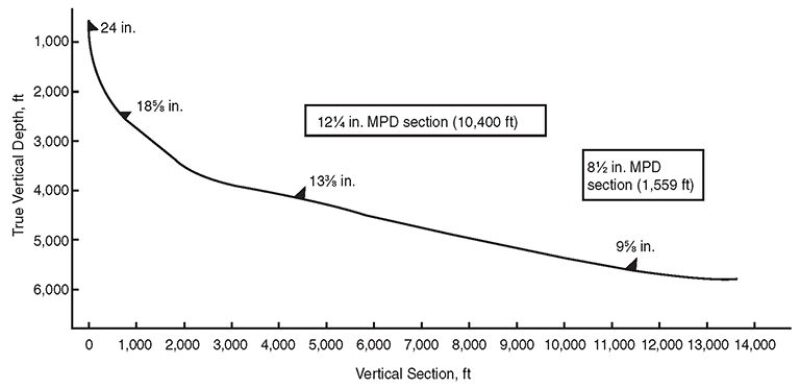
MPD Principles and Benefits for the Harding Field
The basic principle of this form of MPD is to apply annular surface backpressure (SBP) to control the BHP and compensate for annular-pressure fluctuations that result from switching mud pumps on and off. When drilling mud is pumped down the drillstring and up the wellbore annulus, the downhole equivalent mud weight (EMW) increases because of annular friction pressure. This EMW is also known as the equivalent circulating density (ECD). In conventional drilling, an MW needs to be selected to maintain wellbore stability (WBS) and overbalance.
The challenge on Harding ERD wells is that the flow rates required to clean the hole result in ECD values that exceed the sand fracture-gradient limit. In the Harding field, MPD also allows an MW lower than that required for WBS to be used, with WBS being maintained by the additional SBP.
In a standard MPD setup, a rotating control device (RCD) seals the top of the annulus, and the flow of mud from the well is controlled by a choke manifold to apply a desired SBP. The RCD provides a seal on the drillstring while the pipe is moving, allowing the string to be tripped into and out of the well with SBP applied. A limitation of the RCD is that it is reliant on the pipe being tripped having a smooth outer surface with only gradual changes in geometry, and it can seal only within a limited range of diameters. An auxiliary pump is often used to provide flow through the choke, enabling control of the SBP when there is insufficient flow from the mud pumps, particularly during connections.
A further potential benefit of MPD is the reduction of the magnitude of wellbore-pressure variations experienced by the drilled formations during the entire openhole period, thus reducing destabilization and improving the probability of a successful drilling operation.
MPD Project Scope
The project used existing, field-proved MPD systems, processes, and procedures that have been applied successfully on the Harding IC6 well. The goal of the project was to implement a constant-bottomhole-pressure MPD system operating within the physical space constraints and the drilling practices in the Harding field. The team sought to establish MPD as a safe, reliable, and effective method to drill the 12¼- and 8½‑in. sections of the PNE2a well and future Harding wells.
The project scope included the requirement to maintain BHP control during all drilling operations: drilling, tripping, circulating, BHA changeout and cementing, and running screens and liner. It was therefore necessary for the MPD system to operate with multiple fluid gradients to allow cementing and tripping with the FPTP. (For a detailed discussion of the development and testing of the FPTP, please see the complete paper.)
Defining the BHP Window and Mud Weight
Defining the allowable wellbore annular-pressure profile for each of the phases of the well required consideration of the ±50-psi target accuracy. One consideration is that a specific applied pressure is translated as a lower equivalent density the greater the true vertical depth of the point of interest in the well. For example, in PNE2a, the 100-psi reduction in the wellbore pressure window translated to 0.47 lbm/gal at the 13⅜-in. shoe and 0.34 lbm/gal at the 12¼-in. shoe.
Another consideration is that the ECD effect of circulating can be replaced by application of SBP when circulation ceases. However, ECD is annular friction pressure that is distributed along the measured depth (MD) of the well, while SBP has a fixed absolute effect throughout the wellbore annulus. Therefore, the one cannot be directly equated to the other except at one point of interest. For example, if BHP is being kept constant at the bit, it is important to understand the changes to the annular pressure at the shoe when the mud pumps are stopped and started.
The result of this work was that, to avoid exceeding the fracture-gradient limit of the sands, the MW selected for drilling each section was insufficient to maintain WBS in the shales in the event that SBP was lost.
Risk-Management and Operational Procedures
Hazard-identification (HAZID) and hazard-operability (HAZOP) analyses were used throughout the planning of MPD use in Harding. The principal use of the HAZID study was to act as the starting point of a safety assessment in support of the platform safety case and combined-operations safety cases. In addition, it was used to support the operability reviews conducted during the equipment-design process and the operations-risk-assessment process conducted for all MPD well activities.
The principal use of the HAZOP technique was to perform a critical examination of planned or existing processes or operations related to all supplied MPD surface equipment. This included rig and facility interfaces. The HAZOP analysis was chaired by an external process-safety expert.
The written work instructions and rig-contractor procedures used offshore in conventional wells in Harding required modification to incorporate the requirements of the MPD operation. The MPD provider had generic procedures in place that had to be updated to meet the specific constraints of the Harding platform and the PNE2a well.
The decision was made to place company wellsite leaders in the office during the planning phase, and to incorporate the MPD project manager into the core Harding team for the duration of the well. A document package was developed that included MPD rig-up, fingerprinting, drilling, connection, tripping, and contingency procedures.
An underlying principle of the procedures was that the well could be controlled conventionally at all times. Thus, in the event of a well-control event, the well could be safely shut in using the rig’s standard procedures and equipment, effectively isolating the MPD system from the well.
Training was split into two phases, the first being an onshore crew--engagement session that outlined the well design and operational sequence. The session allowed drill-crew and vendor personnel to understand why MPD was being used and how the system would work. The second phase of training was to be conducted offshore with each of the five crews. MPD drilling, tripping, connection, and contingency procedures were practiced in cased hole with a drilling BHA, drilling fluid, and annular-pressure-while-drilling tool operating in order to simulate actual activities as closely as possible.
Ultimately, both the 12¼- and 8½‑in. sections of the PNE2a well were drilled successfully by use of MPD to maintain wellbore pressure within the required WBS/fracture-gradient window. The 9⅝‑in. liner was run to setting depth through the FPTP, past numerous tight spots, and more than 1,900‑ft MD of cement was placed under MPD control without losses. (For a detailed description of the drilling and execution of this well, please see the complete paper.)
Lessons Learned
- Where the MPD tripping strategy involves displacement on the bottom to a low-rheology fluid, a wiper/check trip is recommended to determine the condition of the hole before the displacement.
- MPD-equipment failure contributed over 10 days of nonproductive time. It is important to understand the effects of a loss of any part of the MPD system and to have appropriate backup and contingency plans in place.
- The operation was focused on maintaining wellbore pressures within the target window 100% of the time. It was thought that this was necessary to prevent wellbore instability, but results show that short-duration excursions from the target BHP were tolerated by the formation. These excursions would not have been tolerable had the lower limit on BHP been defined by pore pressure rather than WBS, or if the destabilizing effects of a loss of BHP took place more rapidly than observed.
- The use of the FPTP at high angle and in open hole presented a significant risk that the liner would not be run to planned depth. It was felt that its use was less risky than repeating the use of a more-standard high-viscosity pill, which had allowed mixing of the heavy and lighter fluid columns. Testing and assurance also provided confidence that the FPTP should not destabilize the reactive shales as long as they had previously been drilled with oil-based mud. However, given the issues experienced while running the liner through the pill’s setting depth, further optimization of the liner-deployment strategy would be required as part of the overall well design before use of the FPTP was repeated.
- Use of the MPD system during the cementing of the 9⅝‑in. liner enabled the use of a lower MW to reduce cementing ECDs. It also allowed control of wellbore pressure during the displacement of the cement. Liner cementation was conducted without lost circulation or wellbore instability.
This article, written by JPT Technology Editor Chris Carpenter, contains highlights of paper SPE 166170, “Managed-Pressure Drilling, Casing, and Cementing Enable Success in Conventionally Undrillable Wells in the Harding Field,” by Mohamed A. Mashaal, Tom Fuller, Chris J. Brown, and Robert Paterson, BP, prepared for the 2013 SPE Annual Technical Conference and Exhibition, New Orleans, 30 September–2 October. The paper has not been peer reviewed.