Fall velocities for various plungers have been measured under many different field and simulator conditions. A new theoretical plunger-fall-velocity model uses a specific pressure and temperature for calibration. The model can then be used to calculate fall velocity at other conditions for the same plunger or can be used to show how changing a feature such as plunger weight can affect fall velocity.
Introduction
Conventional plunger lift is a low-cost method for lifting liquids (water, condensate, or oil) from gas and oil wells. Lifting liquids from the well is achieved by closing a surface valve to store energy in the well during a shut-in time period, which is followed by opening the surface valve for a time period so liquids are unloaded as gas flows to the surface. During shut-in, the gas flow is stopped when the controller closes the surface motor valve. The plunger leaves the lubricator to begin its fall from the surface because of a tubing-pressure increase that is caused by closing the motor valve or begun when the plunger is released from a catcher. The plunger falls through gas until entering the accumulated liquid at the bottom of the tubing. Once the plunger is on bottom and sufficient unloading energy is stored, the controller opens the surface valve into the lower-pressure flowline. High-pressure gas in the tubing above the liquid column flows down the flowline, and the high-pressure gas in the casing begins to decrease by expanding to fill the tubing, displacing the plunger and most of the liquid above the plunger to the surface. This plunger-operation cycle is repeated continually to produce the well.
An operator can produce from the well efficiently if the plunger’s fall rate and location and the time taken to fall to the liquid and bottom of the tubing are known accurately. The distance to the plunger and the rate of fall can be determined by examining the acoustic signal created by a falling plunger. The acoustic pulse generated at the tubing-collar recess travels through the gas to the surface to be detected by a microphone, and the change in pressure can be detected by a tubing pressure transducer. These acoustic pulses are normally detected as a plunger falls down the relatively dry tubing interior above the gaseous liquid column at the bottom of the well. Processing this acoustic signal allows the depth and fall velocity of the falling plunger to be determined.
Fig. 1 shows the plunger-fall velocity decreasing smoothly as a function of time. Although there seems to be some scatter of velocities on the plunger-velocity trace, note that the left vertical scale is amplified and that the general trend of the velocity is to consistently decrease as time (plunger depth) increases.
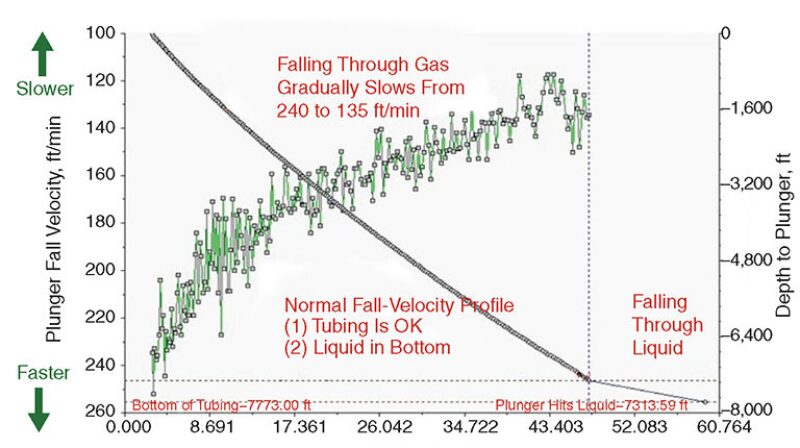
Theoretical General Plunger-Fall-Velocity Model
A new theoretical general plunger-fall velocity model has been developed that can be used to predict plunger-fall velocity in a well at any pressure and temperature condition. If the fall velocity for a particular plunger is measured in a well at a specific pressure and temperature, then the general model is used to determine the calibration constant C. Using a measured fall velocity at a known pressure and temperature to calibrate the general model enables the general plunger-fall-velocity model to calculate the plunger fall velocity at other pressures and temperatures.
Two equations were considered to be representative of the conditions that control the plunger fall through the gas in the tubing. For the first, the “drag model,” the plunger weight is set equal to the drag force acting on the plunger. At a certain pressure, temperature, and gas gravity, the density of the gas is determined. In the “orifice model,” the plunger/tubing seal acts as a choke, allowing a specific amount of gas to be pushed past the plunger as the plunger falls through the tubing.
From field measurements, it has been observed that all plungers fall slower at higher pressure and faster at lower pressure. Field observations during shut-in confirm the general-model predictions of plunger-fall-velocity slowing that is inversely proportional to the square root of the density of the gas through which the plunger is falling.
Plunger-Fall-Velocity Function of Gas or Fluid Density
The data from field testing usually show that the plunger fall velocity decreases as the plunger gets closer to the bottom of the tubing. This behavior agrees with the general plunger-fall model because the increasing pressure increases the gas density inside the tubing.
Fig. 2 shows the measured fall velocity for the same dual-pad plunger in the same 2.375-in. tubing string in the same gas well for five consecutive shut-in time periods. Gas flow into the wellbore from the damaged formation was severely restricted, so for each cycle, the well pressure decreased because the gas inflow did not replace the gas used to unload the well. The average tubing pressure for the five different tests continually decreased. For this padded plunger, there is an almost linear 1.75-ft/min increase in the fall velocity per each 1-psi drop in tubing pressure. When the pressure is low, the plunger-fall velocity is much faster than is considered normal, but when the pressure is higher, the plunger-fall velocity is measured at a rate that is considered normal.
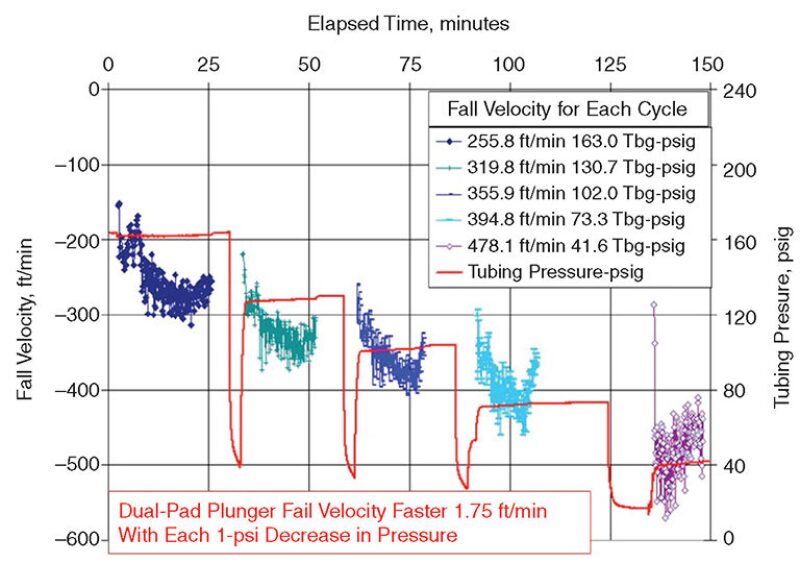
Slow fall velocities caused by high pressure also have an effect on plungers that are considered to fall fast, such as the dual-pad bypass type of plunger. In general, the fall velocity through gassy fluid is approximately 40 ft/min. But when a well is treated with surfactant, the gassy fluid is less dense, and the plunger-fall velocity is near 80 ft/min. When a plunger-lifted well is treated with surfactant, the gaseous liquid column may stand three times taller than a normal untreated gaseous liquid column for the same differential tubing and casing pressure. The plunger falls approximately twice as fast, and the height of the surfactant-treated gaseous liquid column is approximately three times higher. When a plunger-lifted well is treated with surfactant, additional shut-in time may be required to allow time for the plunger to fall to the bottom.
If the plunger becomes stuck in the tubing, all that may be required to unstick the plunger is a fluid level shot down the tubing. The pressure wave from the gas gun discharged at the surface travels down the tubing and often will exert sufficient force to free a stuck plunger. When the plunger gets stuck and does not fall to the bottom, damage to the surface equipment is possible. Dangerous conditions can be avoided when such problems are identified.
Fall Velocity of Different Types of Plungers
As the diameter of the plunger increases, the fall velocity of the same type of plunger decreases. As the diameter of a plunger increases, the weight of the plunger increases. Friction between the plunger and the tubing increases as the diameter increases, because the increase in diameter causes a larger surface area of the plunger to contact the tubing. The cross-sectional area of the plunger increases by the square of the diameter, and less differential pressure is required to resist the fall of the plunger. The larger-diameter plunger increases the friction, and the larger area increases the force from the differential pressure applied to the plunger cross-sectional area, which results in slower fall speed. The presence of pads on a plunger results in slower fall velocities when compared with solid plungers of the same diameter. The slower fall velocity of the pad plunger is caused by the better seal and the additional friction between the tubing and plunger caused by the springs behind the pads. If rubber or neoprene is placed between a set of pads and the plunger body, the improved seal results in a much slower plunger fall.
The bypass type of plunger has a valve that opens to reduce restriction of gas flow past the plunger. The bypass creates a large opening through the plunger, and this large opening allows fluids to flow with less restriction past the plunger. The ability to bypass fluids through the plunger results in higher fall velocity.
Brush plungers have the largest range of fall velocities for any type of plunger. A new brush with soft bristles that fill the area between the plunger and tubing will exhibit a very slow fall velocity (and therefore an efficient plunger). Other brush plungers have stiff nylon bristles that do not contact the tubing wall; this type of brush plunger falls much faster, having a poorer seal. A worn-out brush that appears to be smooth and shiny because of paraffin clogging the bristles, or that has worn bristles that do not contact the tubing, can fall very fast. If a worn plunger is replaced, then the resulting increase in gas production can quickly pay off the cost of the new plunger.
Solid types of plungers that have spiral grooves at the top and bottom of the plunger spin as they fall during shut-in. This spinning motion of the plunger results in slower fall velocities.
Conclusions
Knowing the plunger-fall velocity for specific well conditions will ensure that the plunger will reach the bottom of the tubing by the end of the shut-in period. Use of an acoustic instrument is an effective method to determine the fall velocity during the shut-in time period and provides a calibration point to enter into the general fall-velocity model. Maximum production from the plunger-lift installation will be obtained by having the shortest possible shut-in time equal to the time required for the plunger to reach bottom, as long as sufficient casing pressure exists to return the plunger and accumulated liquid to the surface.
This article, written by JPT Technology Editor Chris Carpenter, contains highlights of paper SPE 164495, “Measured Plunger-Fall Velocity Used To Calibrate New Fall-Velocity Model,” by O.L. Rowlan and J. McCoy, Echometer Company; J. Lea, PLTech; and R. Nadkrynechny and C. Cepuch, T-RAM Canada, prepared for the 2013 SPE Production and Operations Symposium, Oklahoma City, Oklahoma, USA, 23–26 March. The paper has not been peer reviewed.