Carbon dioxide (CO2) waterless fracturing uses liquid CO2 to replace water as the fracturing fluid in reservoir stimulation. The continuity and reliability of the blender are key factors determining performance of the operation. The complete paper proposes a novel closed blender that uses a vertical, rather than horizontal, tanker. This modification can reduce the footprint and effectively suppress CO2 gasification.
×
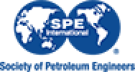
Continue Reading with SPE Membership
SPE Members: Please sign in at the top of the page for access to this member-exclusive content. If you are not a member and you find JPT content valuable, we encourage you to become a part of the SPE member community to gain full access.