The treatment of produced water can be challenging due to variability in both quality and quantity of the produced-water stream. Produced-water quality is dependent on the dynamics and constituents in the oil reservoir, including added injection chemicals and waterflood injection quality. As a consequence of the wide range of variability in both the produced-water quality and the final treated-water quality requirements, it is not easy to determine which technologies are most appropriate for effective treatment and handling—there is no “one size fits all” solution. Varying environmental and regulatory requirements for produced-water treatment as a function of region and location contribute added complexity in decision making. Finding the “best” treatment solution and wading through the multitude of emerging produced-water treatment technologies have become significant challenges for the upstream oil and gas industry.
Considering the current price of oil, there is renewed interest in enhanced oil recovery (EOR) and specifically chemical enhanced oil recovery (CEOR), which holds the potential to unlock millions of barrels of currently unrecoverable oil from offshore reservoirs. However, significant technical and regulatory challenges must be addressed before these techniques can be fully utilized by the industry. Driven by the urgent need for creative, cost-effective produced-water management solutions that protect public and private-sector interests, new treatment technologies are available which offer alternatives to traditional approaches. This is particularly applicable to the treatment of produced water in offshore EOR applications such as CEOR.
Evolving Needs in Produced-Water Treatment
As waterflooded fields mature and continue producing, the amount of produced water to be handled increases, often exponentially. The pressure on operators to manage these larger than anticipated flows of produced water is complicated by the fact that many treatment systems were not designed to handle the higher flows, or to achieve the level of treatment now required by more stringent regulation.
Today, produced water is the highest-volume byproduct associated with oil production, comprising as much as 98% of the total volume produced at the wellhead. Globally, an estimated 70 billion bbl of produced water is generated annually (PFC Roundup, Oil and Gas Facilities, 2012). Of this volume, only about 16% is reinjected (Rahman et al. 2010). In 2008 alone, 19 billion bbl of produced water were discharged into the ocean (Rahman et al. 2010). As environmental regulations are becoming more stringent for offshore discharge, the driver for produced-water treatment innovation is significant, and continues to increase as the industry plans for offshore deployment of CEOR. For example, the northeast Atlantic Ocean currently has a zero liquid discharge (ZLD) regulation in place for offshore production. According to a 2012 study, produced-water reinjection (PWRI) is the only viable technology that can fully address this type of regulation (Rahman et al. 2010).
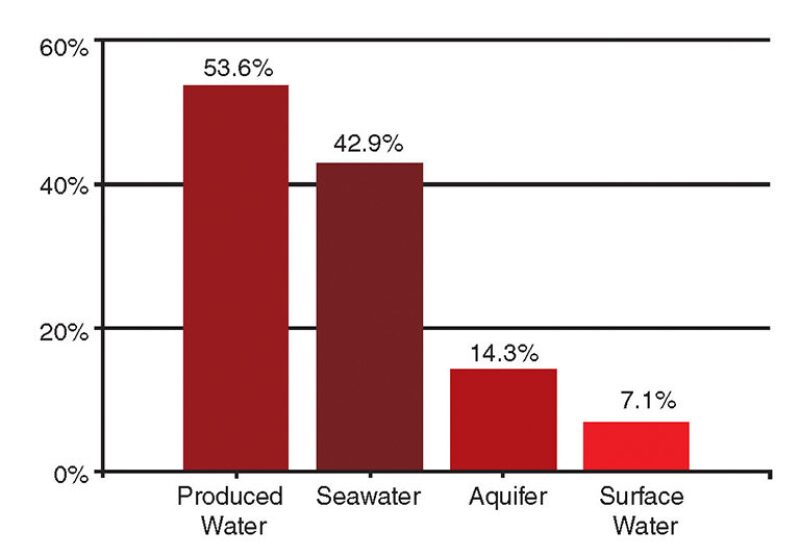
Statistics concur with this finding. A recent survey of operators implementing EOR reported that more than 50% of projects utilize produced water as the source water for EOR reinjection (Fig. 1).
Today, water-based EOR, including low-salinity flooding (LSF) and CEOR, is experiencing increasing interest (Manrique et al. 2010). In evaluating offshore water-based EOR, seawater is generally the most obvious and accessible water source for injection. But, when combining the produced-water regulatory drivers with the growing water-based EOR demands, produced water is expected to float to the top of preferred injection-water sources, if cost-effective treatment is available. Ideally, this water treatment would be highly efficient and able to handle unique aspects of EOR projects, particularly in offshore applications where weight and footprint are more important relative to other features of the treatment system.
Produced-Water Management Challenges in Offshore EOR
Some water-based EOR techniques, such as LSF will have negligible effects on the produced-water stream. But, conversely, a CEOR flood will significantly affect the produced water, and PWRI will be used in these projects. There are numerous produced-water management challenges in offshore CEOR applications, including: 1) technology issues surrounding the influence of CEOR chemicals on conventional produced-water treatment equipment; 2) regulatory requirements and marine environment considerations; and 3) reservoir health concerns when using PWRI. To address these issues, a higher level of produced-water treatment than currently practiced by the offshore industry will likely be required. Each issue is further addressed in the following text.
Technology Challenges
The general differences between onshore and offshore produced-water management strategies are summarized in Table 1. These are important to characterize to fully comprehend the criteria for effective produced-water treatment technologies for offshore applications.
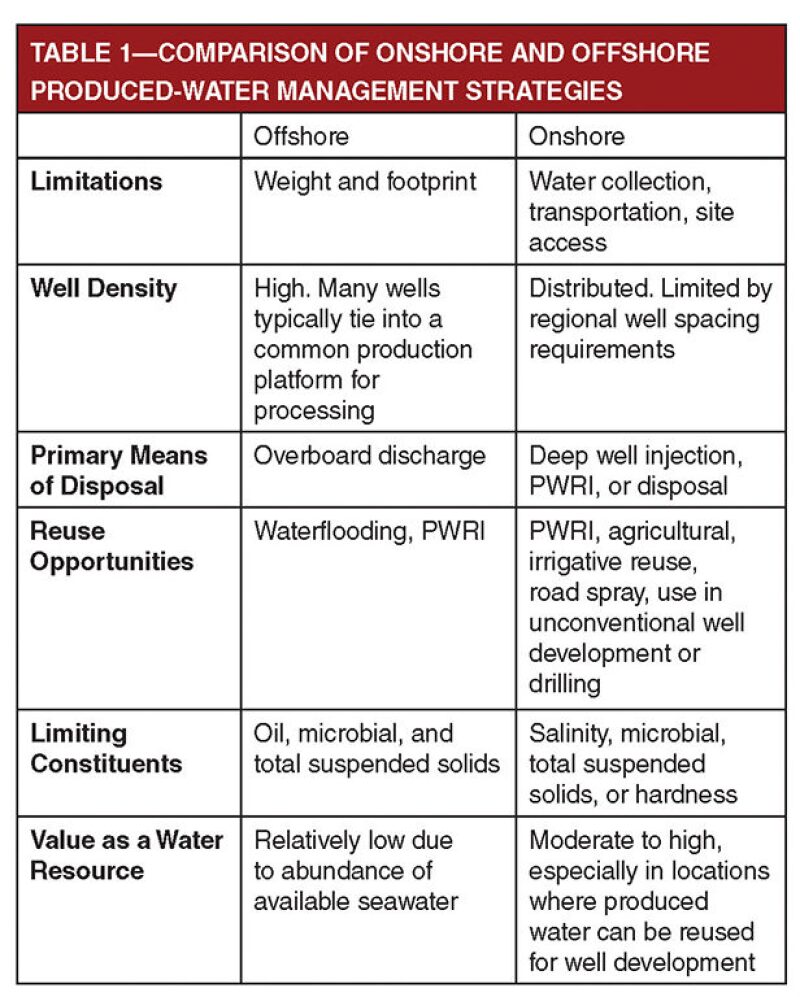
Fig. 2 depicts survey results of produced-water treatment processes used in onshore and offshore EOR projects (Henthorne et al. 2013). Conventional offshore produced-water treatment is most often accomplished by hydrocyclones or gas flotation processes deployed downstream of a three-phase separator. As regulations become more stringent for final oil and suspended solids concentration, or as other forms of produced-water management such as PWRI are more heavily utilized, use of tertiary produced-water treatment for polishing is expected to increase.
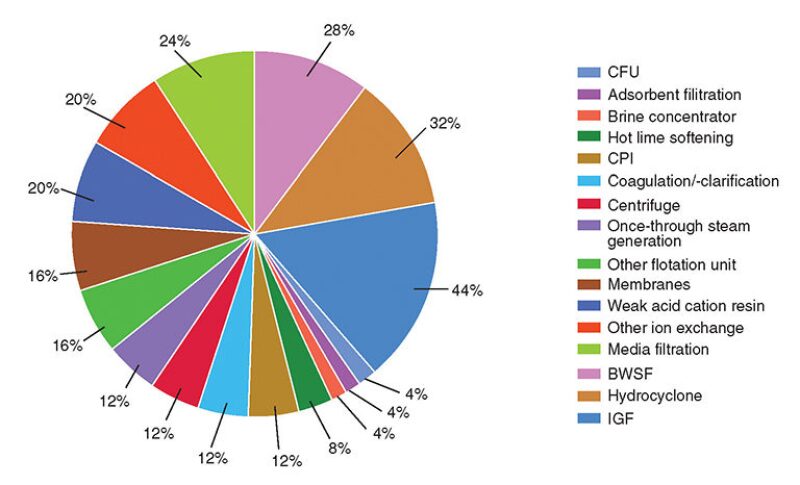
To meet these objectives, conventional black walnut shell filters (BWSF) could be used for polishing. However, although the filters have tested successfully in the North Sea at pilot scale, the number of applications has been limited by technical design challenges related to weight, footprint, and backwash-water requirements. As a result, few full-scale offshore systems have been implemented (Howdeshell et al. 2009). To help address these issues, new polishing technologies are being considered for offshore projects.
Regulatory Challenges
Current regional discharge requirements for offshore produced water are moving toward ZLD, and some regions have already mandated this requirement. The Norwegian Continental Shelf, regulated by the Norwegian Climate and Pollution Agency (KLIF), is moving to adopt an approach in which PWRI availability is targeted at 95% (Stokke and Knapstad 2013; Vik and Dinning 2009). Based on historical operating data provided by Vik and Dinning (2009), many of the systems currently in operation employing PWRI have achieved less than 80% uptime. Only a few of the operators surveyed were able to achieve 95% uptime.
Implementation of some forms of EOR, such as CEOR, can change offshore produced-water management practices. For instance, regulatory agencies have indicated that some alkali, surfactant, and polymer (ASP) CEOR chemicals may be categorized as highly harmful to aquatic environments. With regard to the North Sea, the proposed Oslo and Paris Convention for the Protection of the Marine Environment of the Northeast Atlantic (OSPAR) rules could categorize polymers and surfactant components of ASP as either black or “red” chemicals (Stokke and Knapstad 2013). Under this category and based on proposed risk-based permitting strategies, discharge of produced water containing these compounds would be unacceptable to OSPAR (Aas 2013). Nongovernmental environmental organizations have also become aware of the likelihood of offshore CEOR operations and are sensitive to planned projects.
The siting of injection wells poses another challenge in the North Sea. Under current regulations, offshore produced water can be disposed of via injection into nonproductive formations to comply with Norwegian availability goals. This regulation provides operators with a secondary disposal alternative for CEOR produced water. The use of a nonproducing reservoir also allows the operator to use injection pressures beyond formation fracture pressure. The capability to operate at pressure above fracture pressure helps to ensure water-injection system availability. However, upgrading infrastructure to enable produced-water disposal into a nonproducing reservoir, particularly on older platforms, can be prohibitively expensive due to the cost of drilling additional disposal wells. By comparison, PWRI can be an economically attractive option.
Reservoir Health
If reinjection is the preferred form of produced-water management, project teams must carefully consider produced-water treatment requirements to prevent reservoir damage and oil resource degradation. If produced-water treatment is poor or ineffective, the reservoir may sour, scale, fracture, or plug. Similarly, if the produced water is to be blended with other injection fluids during a CEOR flood, these fluids should be closely scrutinized with regard to blending compatibility and overall blended-water quality objectives to ensure the CEOR flood is successful. If the reservoir loses injectivity during PWRI, the platform could be forced to operate at reduced capacity or shut down until injectivity can be restored.
Typical produced-water treatment approaches target oil and suspended-solids removal. PWRI requires less than 30 ppm oil in water and practical reservoir souring controls to be successful (Vik 2008).
Produced waters can also contain elevated levels of sulfate and organics that can stimulate sulfate-reducing bacteria (SRB) growth in the reservoir during CEOR. SRBs convert sulfate to hydrogen sulfide, which may lead to reservoir souring and health and safety risks for platform operators, or other operational issues. Iron, which is detrimental to polymer performance, can also be present in elevated levels in the produced-water stream. A successful PWRI blending plan must compensate for these additional components during a CEOR flood.
Technologies and Strategies for PWRI in Offshore EOR
Fig. 3 depicts a comprehensive produced-water treatment process that will be driven by economic and regulatory objectives of the project. Fortunately, new technologies and strategies can be used to achieve a suitable water quality for PWRI during offshore EOR applications. When selecting treatment equipment, the impact of CEOR chemicals on conventional treatment equipment should be understood. As a result of anticipated treatment-system performance, a polishing step may be required, especially if PWRI is practiced and when further desalination is required for salinity control or for hardness removal. During a typical project, all identified treatment requirements should be evaluated as well as operation before and after CEOR chemical breakthrough.
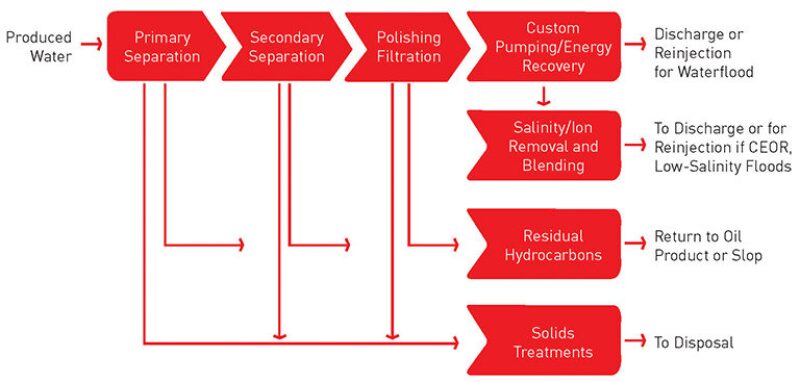
Effects of CEOR on Conventional Offshore Treatment Systems
Two aspects of CEOR chemicals are especially challenging for conventional produced-water treatments systems that rely on gravity separation: 1) the ability of the surfactant to form emulsions comprising small stable oil droplets, and 2) the increased viscosity and non-Newtonian fluid effects from the polymer. An additional effect of EOR and specifically CEOR may be a change in oil properties when oil components that adhere to clays and rocks are released.
Stoke’s law depicts the effects of CEOR chemicals on typical produced-water treatment systems.
The true impact of the CEOR chemicals on the produced-water treatment is experienced after breakthrough has occurred—when residual chemicals and their properties are present in the produced-water stream. The timeframe for breakthrough is highly dependent on reservoir geology, conformance, and well spacing, and for this reason is difficult to estimate during early phases of project development.
The liquid density of CEOR chemical cocktails is controlled by monovalent dissolved solids such as sodium chloride in the CEOR injectant. Polymer, added to improve sweep efficiency, affects viscosity, while surfactant reduces the interfacial tension between the oil and water, forming emulsions. Due to the enhanced oil-droplet and emulsion stability, the performance of the three-phase separator (primary separation) is expected to decrease after CEOR chemical breakthrough.
Three conventional treatment approaches can be employed to improve the performance of the three-phase separator: additional settling time can be provided; production-fluid temperature can be increased; or additional demulsifier can be added. Because of the stability of the oil droplets and likely space limitations of existing platforms, the provision of additional settling time is often impractical offshore. Although acceptable operating temperature will vary depending on the hydrocarbon constituents, an existing separator system will likely have been designed for the anticipated maximum temperature range of the expected production fluid or maximum crude-processing temperature. For these reasons, additional residence time or operation at elevated temperatures may not significantly optimize the performance of the three-phase separator.
The most feasible of the three options is to provide additional demulsifier dosing capabilities to achieve acceptable three-phase separator performance. In addition to production chemicals whose interaction with demulsifier are well understood, selection of demulsifier must consider the anionic properties of the polymer which will generally react with cationic products, the type of surfactant used in the CEOR flood, and the produced-water composition to ensure compatibility and adequate performance in the application (SNF Floerger 2012).
An assumption can be made that readily separable oil and gas components will have been recovered in the three-phase separator. The remaining produced water, which contains potentially elevated levels of relatively stable emulsion, is then treated by a secondary separation process, which will likely include gas flotation, typically induced gas flotation (IGF); compact flotation unit (CFU); or hydrocyclones. Based on SNF Floerger’s reported experience in polymer floods, it is possible to treat viscous produced water, provided the design’s settling velocities or rise rates are calculated using the anticipated viscosity ranges after the polymers have broken through.
IGF and CFU processes rely on gas bubble and oil droplet contact. Coalescing plate interceptors (CPI) can be used as a pretreatment step to encourage the growth of larger droplets for IGF or CFU. The attachment of gas on the oil droplets is expected to increase the rise rate of the oil droplet since the combined mass density of the particle is lower. The combination of droplet and gas is reduced. However, droplet-size modification by the surfactant and increased viscosity from the polymer could largely offset the effect of attached gas in an IGF or CFU, and, as a result, oil-removal efficiency can be affected if CEOR chemicals are present.
The Need for Polishing
After secondary separation, CEOR produced water may require a polishing filtration step to remove fine particles and/or oil in applications where PWRI is performed and especially if salinity/hardness control is needed to improve the performance of the CEOR flood.
In CEOR applications, the filtration load in the polishing process is expected to increase due to the reduced performance of the secondary separation unit. The additional load can have a significant effect, depending on the filter choice and design limits. For this reason, conservative design parameters should be adopted for filter solids loading.
BWSF could provide additional oil removal and has been shown to be compatible with CEOR chemicals in onshore applications. However, the current BWSF systems are not suited to offshore applications, and fine particles and oil droplets will remain in the treated-water stream after BWSF. These particles could be problematic during PWRI and could foul downstream salinity- or hardness-treatment processes. Other treatment technologies, such as specially coated filter media (Narayanan 2013), cartridge filter coalescer systems, and micromedia filtration (Dejak and Sheedy 2013) may provide more effective polishing of smaller oil droplets and fine particles for offshore application.
Ceramic membranes have also been considered for removal of fine particles and oil for offshore CEOR produced water, and their automated washing capabilities are expected to improve operations by eliminating frequent and operator-intensive cartridge-filter changeouts of more conventional technology. Frequent cartridge-filter changeout periods, which typically occur during upset events, can be operationally intensive and tend to generate a large amount of solid waste that must be disposed of at an onshore facility when other forms of filtration are not provided.
Because of the relatively high operating cost associated with cartridge-filter consumption and disposal in an offshore application, enhanced monitoring systems that utilize a combination of fluorescence, spectroscopy, and microscopy will be especially important for CEOR produced-water treatment systems. They provide particle size, differentiate between oil and solids, and identify changes in oil quality. They are expected to be used in many offshore applications, but appear especially well-suited for use in CEOR applications, where they are expected to help optimize operations, especially in cases where polishing filters are provided since they will enable tighter process control that will help to achieve treatment objectives. Where provided in CEOR applications, the monitoring systems should use a self-cleaning procedure to improve their performance and ensure a high level of measurement reliability.
New technologies or treatment processes that use existing technologies in new configurations have been shown to be effective polishing processes. For example, specialized polymeric membranes have leveraged findings from complex industrial water-treatment projects to remove targeted constituents and can be used to recycle polymer. Similarly, electrocoagulation, a process which uses electrical current to destabilize organics, iron, fine particles, and colloids has shown robust performance in CEOR applications. Polymer destruction is also possible using advanced oxidation techniques, and new hyperdense adsorbents are being piloted for offshore CEOR applications.
Concern for Reservoir Souring
The reliance on PWRI is expected to increase as the industry moves to offshore CEOR and resulting ZLD requirements. Critical components of produced-water treatment for PWRI are being identified based on current experience from onshore CEOR projects.
There are several long-term risks connected to PWRI, and the risk of reservoir souring is identified as one of the most important considerations (Garshol et al. 2008). Based on experience with produced-water treatment systems and reported operational issues contributing to downtime in PWRI systems (Fagetbakke 2013), the industry has actively sought secondary biological control measures. An alternative for the control of SRB-induced hydrogen sulfide production in water-oil systems is the use of repeated injections of nitrate. Al-Humam and Gad (2010) reported that the effect of nitrate may cause the presence of chemolithotropic nitrate-reducing bacteria (which not only removes sulfide, but also suppresses sulfide formation by the SRB); competition between SRB and heterotrophic nitrate-reducing bacteria (hNRB) for common electron donors; and the direct inhibition of SRB when nitrite is accumulated during nitrate reduction by hNRB.
Benefits and Opportunities of PWRI During EOR
Although the use of PWRI in offshore CEOR projects is expected to be driven primarily by regulatory requirements, there are numerous potential benefits and opportunities associated with incorporating PWRI into offshore CEOR projects. These include simplification of chemical supply requirements, logistics for CEOR chemicals, a reduction in injectant-treatment system capacity, and regulatory advantages.
Depending on the location of an offshore CEOR project, the supply of chemicals and logistics surrounding injectant production can be reduced considerably through appropriate PWRI. Operators can achieve a net reduction in treatment-chemical consumption by substituting the use of mechanical and thermal processes, such as high-pressure pumps and double-loop heat exchangers, with equipment which will not shear or destroy beneficial polymers (Morel et al. 2010).
Although these techniques may be implemented to conserve polymer, polymer degradation is expected to occur in the reservoir and for other reasons, including the incorporation of iron into the produced water.
The PWRI stream can also be used to greatly offset the treated seawater capacity in an offshore CEOR project by blending prior to injection. The technologies are now available to tune, or customize, the treated water to compensate for the PWRI water chemistry and to ensure that the blended injection stream meets the CEOR-cocktail water chemistry requirements. The availability of the PWRI can thereby significantly reduce the seawater treatment capacity.
Summary and Conclusions
It is not surprising that oil and gas companies considering offshore CEOR are also considering PWRI as their primary form of produced-water management since it may be the most efficient and environmentally friendly option available.
Where PWRI is implemented, significant planning is required to ensure that the produced-water treatment system is capable of treating to high enough quality to maintain reservoir health. Implementation of new and emerging technologies is expected to enhance the appeal of PWRI, such that the industry will come to recognize it as a resource rather than an operating cost.
The impact of CEOR chemical properties must be considered in produced-water treatment, both for existing and upgraded treatment equipment and chemical options. Often, these effects are the last to be considered and may lead to schedule delays. Although the uncertainty and complexity of the produced-water stream is a barrier to implementation of offshore CEOR projects, the use of PWRI holds potential to resolve environmental hurdles, reduce seawater-treatment system capacity and ASP chemical consumption. For these reasons, advancements in produced-water treatment that facilitate PWRI technologies may be the project enablers that will lead to global implementation of offshore CEOR.
References
- Aas, N. 2013. Assessment of Environmental Impact from EOR Chemicals on the Norwegian Continental Shelf. Paper presented at TEKNA Produced Water Management conference, Stavanger, Norway.
- Al-Humam, A. and Gad, M. 2010. The Mechanisms of Nitrate Action on SRB in Sulfate-Nitrate Switching using Molecular Microbiology Techniques. Saudi Aramco Journal of Technology Summer 2010.
- Dejak, M. and Sheedy, M. 2013. Performance Testing of Micromedia and Conventional Filters Operating on Heavy Oil Produced Water in the San Joaquin Valley (California). Paper presented at the 23rd Annual Produced Water Seminar, Houston, Texas, USA.
- Fagetbakke, A. 2013. Grane PW Injection in Trouble. Paper presented at TEKNA Produced Water Management conference, Stavanger, Norway.
- Garshol, F., Schwermer, C., and Dinning, A. 2008. Microbial Impact Control in Offshore Water Systems. Exploration & Production Oil & Gas Review 6(11): 68–71.
- Henthorne, L., Walsh, J., and Llano, V. 2013. Water Management for EOR Applications—Sourcing, Treating, Reuse and Recycle. Paper OTC 24199-MS presented at the Offshore Technology Conference, Houston, Texas, USA, 6–9 May.
- Howdeshell, M., Feltch, C., and Lorge, E. 2009. Walnut Shell Filters: Redesigning for Offshore Use. Paper presented at TEKNA Produced Water Management conference, Stavanger, Norway.
- Manrique, E., Thomas, C., Ravikiran, R., et al. 2010. EOR: Current Status and Opportunities. Paper SPE 130113-MS presented at the SPE Improved Oil Recovery Symposium, Tulsa, Oklahoma, USA.
- Morel, D., Vert, M., Jouene, S., et al. 2010. First Polymer Injection in Deep Offshore Field Angola: Recent Advances on Dalia/Camelia Field Case. Paper SPE 135735 presented at the SPE Annual Technical Conference and Exhibition, Florence, Italy.
- Narayanan, A. 2013. High Oil Recovery from Produced Water Streams Generated through EOR Process. Paper presented at the 23rd Annual Produced Water Seminar, Houston, Texas, USA.
- Produced Water Treatment: Yesterday, Today, and Tomorrow. 2012. PFC Roundup. Oil and Gas Facilities 1(1): 29–30.
- Rahman, et al. 2010. The Offshore Produced Water Gamechanger Report 2010–2014. Douglas-Westwood Limited, Canterbury, UK.
- SNF Floerger. 2012. Enhancing Polymer Flooding Performance: 30 Years of Experience in CEOR. Altavia, St. Etienne, France.
- Stokke, R. and Knapstad, H. 2013. EOR and Environmental Concerns. Paper presented at TEKNA Produced Water Management conference, Stavanger, Norway.
- Vik, E. 2008. Reducing the Impact of Discharges to Sea. Paper presented at the OG21-TTA1 Seminar at Norges Forskningsråd, Oslo, Norway.
- Vik, E. and Dinning, A. 2009. Produced Water Re-Injection — The Potential to Become an Improved Oil Recovery Method. Exploration & Production Oil & Gas Review 7(1): 1–3.
About the Authors
Buddy Boysen is a process engineer with Water Standard and its produced-water product champion. His experience includes advanced water-treatment system testing, design, commissioning, and operational support of municipal and industrial water, wastewater, and reuse facilities, and engineering and operational support of onshore produced-water management facilities.
Lisa Henthorne is senior vice president and chief technology officer with Water Standard, focusing on developing customized water chemistries to enhance oil recovery in low-salinity and chemical enhanced oil recovery.
Holly Johnson is a process engineer with Water Standard, experienced in designing and developing advanced water-treatment solutions for industrial and municipal clients worldwide.
Becky Turner is a business development and marketing coordinator with Water Standard, where she helps manage technical proposal development.