This paper investigates novel approaches to sour-gas treatment for use in the Middle East that are outside the common oil and gas market and compares them with traditional techniques. The methods presented in this paper were based on one central tenet: deliver a practical, economical solution to producing from an extremely sour [greater than 40,000‑ppm hydrogen sulfide (H2S)] gas field. Modified tail-gas treatment units (TGTUs) were found to be economically viable, can be implemented onsite, and can tie in to existing infrastructure with minimal capital expenditure (CAPEX) or operational expenditure (OPEX).
Introduction
A field in the Mediterranean was producing sour gas at a rate that would render production untenable. One of the wells was producing 24,000-ppm H2S with an expected increase to 46,000 ppm within a few years.
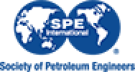