Current reservoir-modeling work flows are rigid, because modification to the understanding of the underlying structural model often requires a complete regeneration of the reservoir grid, which brings additional costs, delays, and incompatibilities with past calculations. This paper proposes a novel work flow for structural-features modeling that allows the introduction of faults and other structural and nonstructural features to any simulation grid without modification.
Introduction
The modeling of hydrocarbon reservoirs is a multiscale and multidisciplinary process that usually involves several months for advancement from seismic interpretation to reservoir simulation. One crucial moment in the life of a reservoir model is the creation of its reservoir-simulation grid. This involves the encoding of the so-called structural model (Fig.
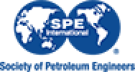