The complete paper discuses a well with a history of sand production that exhibits long cyclic slugging behavior. Whether the slugging is caused by the gap at the well’s lower completion, by sand transportation, or by both is not fully understood. Dynamic wellbore modeling with sand-particle transport is essential in modeling the complex slugging behavior. Transient simulations successfully produced the slugging behavior observed in the field.
×
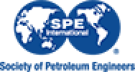
Continue Reading with SPE Membership
SPE Members: Please sign in at the top of the page for access to this member-exclusive content. If you are not a member and you find JPT content valuable, we encourage you to become a part of the SPE member community to gain full access.