Plans were developed to produce multiple deepwater reservoirs with vastly different fluid properties from a subsea development in 3,100 ft of water in the Gulf of Mexico. Diagnostic and flow-assurance (FA) testing of the different-density oils addressed viscosity including non-Newtonian, wax, asphaltene, and hydrate behavior; downhole and flowline commingling; and fluid-compatibility tests. Provisions for pigging and a chemical-treatment program were added for extra assurance. A larger-sized oil-export line was selected to allow sufficient capacity for blended or heavy oils after restart.
Introduction
The Who Dat field is in the Mississippi Canyon protraction area of the Gulf of Mexico and is being developed with the Opti-Ex semisubmersible floating production system (FPS), which has a capacity of 60,000 BOPD and 150 MMcf/D of gas. The field primarily contains oil and consists of ten stacked amplitude-supported reservoirs in a salt-withdrawal minibasin. Three wells had been drilled at the time this paper was written, penetrating more than 700 ft of net pay in nine distinct reservoirs ranging in depth from 12,000- to 17,000-ft true vertical depth. Both gas and oil reservoirs were found, with varying fluid properties. Significant fluid data were acquired in the openhole program, with more than 60 downhole fluid samples. Twelve full pressure/volume/temperature (PVT) studies and eight diagnostic FA studies were performed to gain an early understanding of the fluid. Later, flowback oil samples were acquired, rechecked, and evaluated further.
Field development includes 12 subsea wells (i.e., wet trees) flowing into three four-slot subsea manifolds. Drill Center A has one manifold, and Drill Center E has two manifolds. Each manifold is connected to the FPS with dual 6-in.-nominal-diameter wet-insulated flowlines and flexible risers having the capacity of roundtrip pigging. These infield flowlines are approximately 3 miles long, and the seafloor geometry results in a downsloping flowpath. The project includes a 10-in. gas-export line and a 14‑in. oil-export line, also with flexible risers. Fig. 1 shows the subsea layout.
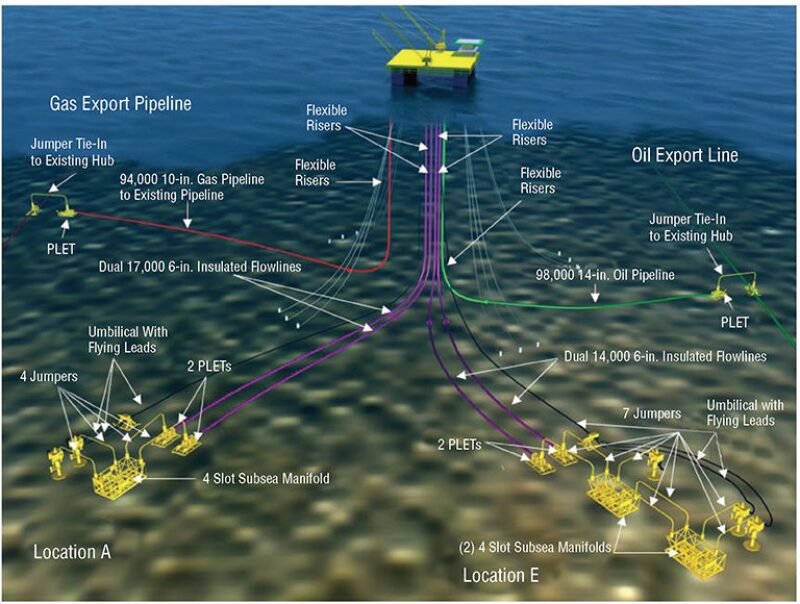
The key inputs for the FA process are uncontaminated fluid samples and detailed laboratory analysis. In the case of system selection for the Who Dat field, fluid samples were available from three wells and multiple formations. There were uncertainties about key formation-fluid properties resulting from drilling-fluid contamination, including viscosity, foaming, and wax content.
Varying Fluid Properties and FA Diagnostics
A steady-state model was constructed early in the appraise/select stage to link the reservoir tanks, variable fluids, well productivity, wellbores, flowlines, and platform locations. This model, along with transient models, was used to confirm the hydraulics and FA of various production-system options. Because of the FA challenges (mainly viscosity) of some of the producing formations, some options were eliminated—namely, new platform builds in shallow shelf waters requiring long subsea-tieback options.
FA Testing and Challenges
The rather high wax content in some of the oils raised concerns of potential wax-deposition problems. Also, a wax-crystal formation rate in Zone RG of 0.03 raised the possibility of wax problems. Oils from two of the key sands, RG and RE, were selected to perform cold-plate tests to determine wax-deposition tendencies. The data were used to calculate the pigging rate required for the worst-case scenario of flowing 10,000 B/D of RG oil alone in the 6-in. 17,000-ft flowline without insulation and chemicals. The required pigging rate was estimated to be 996 days without chemical treatment and 3,984 days with a 50%-effective chemical treatment. Considering that the flowline is insulated and chemically treated, it was appropriate to conclude that no serious wax issue should be expected from the RG oil. All other oils have less of a wax issue.
Viscosity Data. The high viscosities alter the hydraulic behavior of the oils from Newtonian to non-Newtonian. Some of the oils—namely, from Sands RF and RE—fall into this category. Viscosity is a function of only temperature, pressure, and composition, not a function of flow rate (or shear rate) or past fluid history (either thermal or shear history). The viscosity of a Newtonian fluid is independent of applied shear forces. Waxy crude oils at temperatures approaching their pour point behave more like pseudoplastics or ideal plastics. In some oils, the flow behavior of the fluid is non-Newtonian. In other words, the viscosity of the fluid is a function of the shear rate (or flow rate). Non-Newtonian fluids without a yield stress at all ordinary temperatures are called pseudoplastics or dilatant plastics, which are in the category of power-law fluids. There is evidence that the flow behavior of RG and RE oils is slightly non-Newtonian (namely, pseudoplastic).
Commingling Compatibility. No incompatibilities were found when mixing the oils. It was found that commingled production of some of the oils could alleviate viscosity issues. No major FA issues were found with the Who Dat reservoir oils in a 3-mile tieback development, other than hydrates. There is a slight wax-deposition issue with the RG sand, and viscosity issues with the RF and RE sands were deemed not to be a serious FA threat to the project at this tieback distance.
FA Plan and Challenges
The FA risk and concerns were identified through the project-design phases: feasibility studies, tieback options, front-end-engineering design, detail design, and operations. After performing extensive fluid testing and PVT analysis for each reservoir, various thermohydraulic models were developed with a transient model. The models included wellbores, infield flowlines, and an integrated production system. A steady-state analysis and a transient hydraulic analysis of the production system for the expected operation and operating condition were key parts of the Who Dat FA study. However, the details of this work are not presented in this paper. The FA plan presented here refers to the issues associated with solid deposition, liquid management, and producing high-viscosity fluids.
In Drill Center E, the wells will be predominantly oil wells until midlife gas zones are completed. However, there is significant fluid-phase dynamics regarding the Drill Center A wells early on. In one potential scenario, Sand RG is initially an oil reservoir that may become a gas producer. Various oil-production strategies were studied.
Steady-State Production. The basic production strategy for steady-state production is to use wet insulation and maximum production or commingled production to prevent hydrate formation in the subsea production system and to prevent wax deposition in the flowlines. The wells produce at rates controlled with the combination of subsea and topside choking. During normal steady-state production, the subsea-choke openings are maintained to minimize the subsea pressure and temperature losses and to maintain optimal steady-state-flow characteristics in the flowlines.
Hydrate Management. During steady-state oil production, the primary hydrate-prevention method is to keep the flow rate high enough to maintain the flowing temperature outside of the hydrate region. The following design and operational philosophy was adopted.
- Thermal insulation on flowlines and risers will provide sufficient cool-down time
- For a long-term unplanned shutdown, flowlines and risers can be depressurized and displaced if necessary
- Methanol injection into the subsea system during startup and shutdown
Fig. 2 shows the early-life steady-state-production pressure and temperature profiles for various reservoirs and producing rates. In all cases, the choking at the seafloor adds heat (5–10°F) and can be managed when necessary. The hydrate curves are superimposed on Fig. 2 and show that operations are expected to be out of the hydrate region in steady-state conditions.
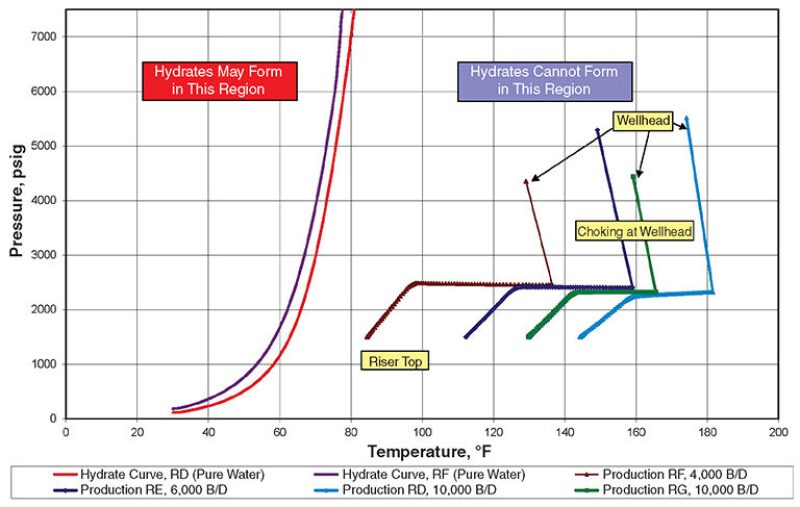
Slugging/Liquid Management. The downsloping bathymetry leading to the riser provides a propensity for slugging at the top of the riser. Slugging can be hydrodynamic in nature, with liquid-arrival rates oscillating but with negligible surge volumes, or can be terrain-induced slugging characterized by large slugs of liquid followed by periods of no liquid flow. Both types of slugging were investigated for the production system.
Early- and late-production profiles were evaluated to determine the expected point in time for which each scenario could become an operational issue. Because Who Dat wells produce through a multiple-riser system, the slug-handling capacity is likely shared with other producing wells (i.e., other wells’ production decline may vary over time). The flowline route from Drill Center E to the host has a low spot near the riser base, which could make the system prone to slugging.
However, terrain-induced slugging is expected once arrival pressures drop to low-pressure conditions, because gas breakout will occur in the down-sloping portion of the flowline resulting in slug initiation at the riser base. On the basis of the hydraulic results, flow instability may occur in later life, after flow rates drop below 5,000 B/D for a single flowline.
Gas Lift Provisions. Early thermal/hydraulic studies identified gas lift being required in late-life production because of the hydrostatic pressure in the riser at this water depth and the downward-sloping flowpath from the drill center. The need for post-installation gas lift will be assessed after sufficient production history is gathered. If needed, the gas lift system could be used for riser unloading, enhanced startup, or continuous injection to aid production-flow-rate improvement.
Operational Issues and Chemical Program
The subsea-production-system operating strategies were designed to prevent the formation of hydrates and prevent deposition of paraffin in the subsea production system during all operation situations. The insulation capacity of the flowlines was selected to maintain the temperature of the produced fluids above the produced-fluid wax-appearance temperature (WAT) during most of the well-production life at base-case production conditions. When the temperature of the produced fluids in a flowline falls below the WAT, continuous injection of paraffin inhibitor is planned.
Wax Control and Remediation. In practice, paraffin inhibitor is injected continuously at the subsea wellheads to provide wax inhibition for the oil-export pipeline. Whereas the flowline insulation may protect the well-to-FPS flowlines from paraffin deposition, paraffin-inhibitor injection is required to protect the uninsulated oil-export pipeline. It will be more cost effective to use the subsea chemical-injection system continuously and share the operating costs of the oil-export pipeline.
Hydrate-Formation Control. The operating strategies are designed to eliminate hydrate formation in the subsea flowline systems. During steady-state production operations, the produced fluids in the flowlines are maintained sufficiently warm by the flowline thermal-insulation capacity, to prevent the formation of hydrates at the high flowing-system pressures. For emergency shutdown and process shutdown, wells are shut in by initially closing the topside flowline-shutdown valves and allowing the flowlines to pressure up to the full shut-in wellhead-system pressure. For emergency shutdowns, the tree production wing valves are closed subsequently to isolate the tree from the flowline. The insulating capacity of the flowlines is sufficient to maintain the temperature of the produced fluids in the flowlines above the hydrate temperature for the shut-in pressure conditions for a long time period after the shut-in, which allows the shut-in condition to be corrected and production restarted.
Initial Startup. All flowlines were left filled with filtered seawater after installation and hydrotesting activities. The flowlines were dewatered with produced fluids back to the platform during startup by processing the water through the topside process-handling equipment and then discharging the water overboard. Before startup, 1,000 gal of methanol was injected into each flowline to provide a hydrate-inhibiting buffer between the seawater and the produced hydrocarbons. An additional 350 gal of methanol was injected into each well to equalize the pressure across the surface-controlled subsurface safety valves (SCSSVs) and allow these nonself-equalizing valves to open. These steps were taken immediately before flow was initiated, to minimize the amount of methanol absorption into the seawater-filled flowlines.
Restart. The basic strategy for restart of the production system is to get as much heat as possible into the subsea system and flowlines quickly, to move out of the hydrate region. This method is contingent on the flowline-system shut-in conditions. The areas of concern during restart are the SCSSV, trees, jumpers, and flowlines. Because there is no downhole injection for the hydrate inhibitor, the wellbore above the SCSSV must be bullheaded with 350 gal of methanol. Methanol will be injected upstream of the subsea choke at a rate of 5 gal/min, which will inhibit the flowline through flow of the well. Methanol injection upstream of the subsea choke will continue until the flowline system moves out of the hydrate region.
Thermal Performance (Prediction vs. Real Data)
During the initial startup of the Who Dat Well E-2, the thermal performance of the flowline system was benchmarked with the well-flowback data. The thermal performance of the system is better than expected. This performance may result in a longer-than-expected cool-down time, and it may provide the operation team more flexibility.
Export Pipeline
A large oil-export line (14 in.) was selected to allow sufficient capacity for blended or heavy oils. When pipeline flow is restarted with cold crude, the viscosity is high and there is a possibility of insufficient pressure (pumping energy) to generate enough flow velocity in a long pipeline to shear the crude and develop lower viscosities. Additionally, high oil-export-pipeline pressures can occur if the oil-export line is full of only heavy oil. When this occurs, even though the gel is broken, the flow rates will remain very low until the high-viscosity oil is displaced with a blend. A future operating consideration when exporting the heavy oil is to use more-costly chemical solutions to prevent gel formation and high export pressures.
This article, written by Senior Technology Editor Dennis Denney, contains highlights of paper OTC 23378, “Subsea Who Dat Project—Producing Light and Heavy Oil in a Deepwater Subsea Development,” by Gerard J. Simms, SPE, Bruce Cooley, SPE, and Rick Fowler, SPE, LLOG Exploration; Kosta J. Leontaritis, SPE, AsphWax; and Kana Krishnathasan, SPE, Intecsea, prepared for the 2012 Offshore Technology Conference, Houston, 30 April–3 May. The paper has not been peer reviewed. Copyright 2012 Offshore Technology Conference. Reproduced by permission.