This paper presents the first successful application of ceramic sand screens offshore Malaysia. Ceramic sand screens were considered as a remedial sand-control method because of their superior durability and resistance compared with metallic sand screens. Remedial sand control with ceramic sand screens has successfully revived idle wells back to production at a lower total cost, with oil gain beyond the initial target and a higher return on investment.
Field Overview
The field was discovered in July 1967 at a water depth of 250 ft below mean sea level approximately 40 km offshore East Malaysia. The structural configuration of the field is characterized by a simple, low-relief domal anticline resulting from the rollover associated with regional growth faulting.
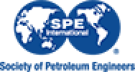