Wells often require being drilled through and cemented across salt formations. In many parts of the world, salt sections consist of multiple salt types. This analysis shows that intercalated salts subject cement sheaths to a series of tensile and compressive loads whose magnitude depends on the size and relative position of different salts. The salt/salt-interface effects dominate the general tenet of increasing creep rate with increasing depth.
×
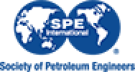
Continue Reading with SPE Membership
SPE Members: Please sign in at the top of the page for access to this member-exclusive content. If you are not a member and you find JPT content valuable, we encourage you to become a part of the SPE member community to gain full access.