Pressure/volume/temperature (PVT) phase behavior characterization and geochemical compositional analysis of petroleum samples play a crucial role in the determination of producible reserves and the best production strategy. Openhole samples are the most-valuable types of samples for PVT and geochemical analysis but are costly and limited to 10 to 20 samples. The complete paper presents a technical discussion of a new microsampling technique for logging while drilling (LWD) and a corresponding wellsite technique to provide compositional interpretation, contamination assessment, reservoir-fluid compositional grading, and reservoir compartmentalization assessment. This microscale approach enables fast analysis by using field or near-field deployment of the analytical tool.
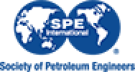