Interactions between fractures in adjacent horizontal wells and development of methods for mitigating their costly negative effects has become the focus of much discussion and debate within the fracturing community. The impetus for this attention has been the impact of these interactions on productivity and mechanical integrity of these wells. Faced with these challenges, a group of 15 subject-matter experts representing different parts of the fracturing community held a 2-day meeting in December 2018 for a very open and confidential discussion of the technical foundation of the various aspects of the subject: what are the root causes of these interactions, their types, consequences, industry experience with various mitigating actions, and what has worked and what has not. At the conclusion of the meeting, the group decided to prepare a report of its deliberations and make it available as a service to the industry.
Soon after the start of the discussions, the group recognized the multiplicity of terms used for each of the various aspects of the subject. Furthermore, these terms were viewed to be vague and without a descriptive technical foundation. Having been developed during the early days of discovery of these events, they generically identified them as problems with minimum regard to the technical roots of their occurrence, variations in their types, or cause-and-effect relationships that may exist between the type of events and their impact on well productivity or mechanical integrity. Development of proper technically-based terms and their definitions were the first outcome of the group’s deliberations. The terms defined below are reflective of the relevant applicable technologies and are intended to be descriptive of the physical events that occur during these events that control their behavior. It is the group’s hope that use of these terms will increase clarity and accuracy to the extensive technical content currently devoted to this subject.
To start with, the term “frac-driven interaction (FDI)” was recommended for defining the broader overall subject. This term is offered as replacement for “frac hit,” “frac bashing,” “frac intersection,” etc., all of which have a negative connotation. It was argued that a neutral term is more suited for describing these events, since all FDIs do not harm adjacent passive wells. Some of them can in fact provide valuable information about engineering characteristics of the created fractures and detecting their existence and identifying their type can serve a useful purpose by offering the information needed for the next generation of fracturing treatments. Additionally, the term “frac hit” projects an unnecessarily negative impression to the public, environmental groups, and state and federal regulating agencies.
The definitions offered to identify the status of wells and fracturing are listed below.
Active well. The well where fracturing (or refracturing) operations are currently taking place.
Passive wells. Wells offset to the active well. These wells may be shut in and their pressure monitored for diagnostic purposes. In practice, these wells are often already fractured.
Primary well. This is the first well drilled for production in a given area that is not within the depletion zone of another well. This well is sometimes referred to as the “parent well.” The technical importance of the recommended designation is related to the fact that production from a well reduces the reservoir pressure within its depletion zone, which in turn reduces the magnitude of the principal stresses. Lower principal stresses attract approaching hydraulic fractures from adjacent wells.
Infill well. This is a well drilled for production in the same area that is located within the depletion zone of the primary well or other infill well(s). This type of well is sometimes called “child well.” The technical importance of this designation is related to the fact that principal stresses on the two sides of this well are not the same. Their magnitude gradually reduces in the direction toward the primary well, and increases away from it. This definition also applies to a well drilled for production offsetting a primary (and/or other existing well(s)) whose targeted pay interval overlaps the primary or other existing well(s).
Active fracture. Currently growing fracture with its fluid pressure above the minimum in-situ principal stress.
Passive fracture. The previously created, currently closing or closed fracture that may or may not be somewhat pressure depleted.
Frac Stage. A single pumping event into an isolated section of the well where fluid can exit the casing from one or more discrete points (perforation clusters or sleeves) separated by a specific distance.
Cluster. A closely spaced group of perforations that are intended to provide slurry conduits for creation of a single fracture.
Fracture interactions. This term defines the effect of each active or passive fracture on the propagation of other fractures in its vicinity, and is divided into two general groups:
- Intra-well interactions. Interactions between active and passive fractures within the same well.
- Inter-well interactions. Interactions between fractures in offsetting wells.
Stress shadowing. The stress alterations caused by local and accumulative deformations of the formation caused by existing fractures in the formation. The magnitude of stress shadow depends on the elastic properties of the formation, the length and width of the closing/closed passive fracture(s), and the separation between active and passive fractures. Since the dimensions of closing fracture(s) change with time, the magnitude of stress shadow is also time-dependent until closure of the passive fracture(s) is arrested by proppant.
Dynamic active fracture interactions (DAFI). The interaction between multiple, simultaneously growing active fractures. As these fractures grow, they alter the state of stress within the surrounding formation, which may cause deviations in the orientation of each active fracture and their required common extension pressure. By definition, there is no DAFI if only a single fracture is created during each pumping stage.
Fracture shadow. The high fluid pressure inside an active fracture(s) causes compaction of the surrounding formation and compression of the fluid inside passive adjacent closing/closed fracture(s). This increase in the passive fracture(s) fluid pressure is transmitted to the wellbore it is connected to, and where it is also recorded. The magnitude of the pressure change depends on the active fracture extension pressure and geometry, elastic properties of the formation, distance between fractures, and the total volume of the passive well and the fractures to which it is connected. Once the active fracture stage is completed, the magnitude of fracture shadow slowly decreases as the active fracture gradually closes with time. A residual long-term pressure increase corresponding to the closed previously active fractures will continue to exist in the passive well (especially if the fracture is propped open). An increase in formation fluid pressure may also contribute to fracture shadowing, but this has a much smaller contribution than the elastic effect in ultra-low permeability and low-porosity unconventional reservoirs.
Fracture shadowing does not involve exchange of fluid between adjacent fractures and is not likely to cause production or mechanical damage to offset wells.
Frac/frac connections. Some level of fluid migration occurs between adjacent fractures in these types of interaction. Based on observed field data, these can be divided into two categories: temporary and long-term.
- Temporary frac/frac connection. This term defines the temporary hydraulic link between created fractures or wells during active fracturing that disappear at the end of each active fracture stage. Fracture pathways that create these links close once the corresponding fracture stage is completed and the fractures and/or contacted natural fractures have closed. While fracturing, there is fluid migration into the passive well during these types of FDIs.
- Long-term frac/frac connection. This term defines a hydraulic link between fractures or wells that continue beyond the end of active fracturing. Fracture pathways (induced and/or natural) that create these links are conductive, stay at least partially open after fracturing, and are likely to result in long-term fluid exchange between the two wells and production interference. They can also cause mechanical damage to the passive well.
Bounded well. A well with another well on each side of it.
Partially bounded well. A well with a well only on one side of it. There is no well on the other side within its depletion zone.
Unbounded well. Does not have another well on either side.
Pre-loaded well. A producing well that is offset to the new infill wells that has been shut in and its pressure has been increased by injecting fluid (usually water) into it.
Defensive frac. A fracture created by injecting fluid (usually water and often no proppant) in a producing well that is offset to the new active infill well.
Technical Terminology
While the group was aware of the fact that some of the old terms have become entrenched within the fracturing community, it was hoped that awareness of the alternate terms offered here will gradually encourage switching to more technically based terminology that will give the subject a stronger technical backbone and help clarity of the discussions.
Identification of the type of FDI is important for establishing if the event can harm the adjacent passive wells. Pressure data monitored in these wells can easily indicate the type of FDIs induced between adjacent wells in real time. Some types of FDIs are, in fact, benign and do not cause mechanical or production damage in adjacent wells. For example, fracture shadowing and low-intensity temporary frac/frac connections are not likely sources for the types of production or mechanical damage that require real-time mitigating actions. Furthermore, careful analysis of these types of interactions can reveal valuable information about the created fractures, including estimates of their length, orientation, height, conductivity, etc. High-intensity temporary or long-term interactions are the more likely sources of the types of costly damage that is of most concern to the fracturing community. Case histories supported by field data were used in the meeting to show the different types of FDIs actually encountered in field operations and their identifying features.
In the ensuing discussions, FDIs were divided into two general groups, intra-well and inter-well. For each case, the discussions covered the influence of formation properties, in-situ stress magnitudes, type and intensity of natural fractures, completion integrity including effectiveness of isolation between different fracture stages, type of completion, fracture treatment design, and other parameters on the type and intensity of FDIs. The discussions were aided by actual field data.
In the next part of deliberations, participants presented their own experiences with various types of FDIs and the type and effectiveness of the various operational steps they had taken for mitigating their negative effects, their practical considerations including costs and simplicity of operations, etc. In general, these actions were classified as: do nothing, shut in the offset passive well, long-term shut-in, frac and flow, small pre-loads, use of diverters, high-rate water defensive fracs, and re-fracs. Specific features of each of these actions, their effectiveness, and the critical parameters for ensuring their success were discussed in detail.
The group recognized that detection and identification of the type and intensity of FDIs is the key to the next generation of fracturing operations. The presence of FDIs can easily be detected in real time by monitoring the fluid pressure in adjacent shut-in passive wells. Measurement and analysis of this data can provide a valuable guide to deciding whether continuation of the fracturing treatment has the potential to harm the passive wells. In case of risk of damage, the operator can decide whether to discontinue the present fracturing stage, alter the in-progress active well fracture simulation, or to continue while executing mitigation steps in the passive wells.
At the end of these discussions, the group charged the authors of this article to prepare a report of the public part of the discussions, including an up-to-date list of applicable publications, and make it available to the fracturing community. The full report is available in the Hydraulic Fracturing Journal. This issue also includes three other papers written by meeting participants that supplement the general aspects of the subject. These papers present the critical parameters that influence success of mitigation operations, casing deformations and failure in fractured horizontal wells, and methods for real-time monitoring pressures in the primary and in-fill wells.
Copies of the journal can be purchased online at http://petrodomain.com/frac-driven-interactions.
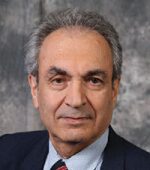
Ali Daneshy, SPE, is president of Daneshy Consultants International. His fracturing experience spans 50 years during which he has published numerous technical papers related to the subject. He is an SPE Honorary Member, SPE Distinguished Member, and received the SPE Distinguished Service Award for his contributions to hydraulic fracturing. He also teaches industry short courses related to fracturing. He holds an MS in mining engineering (rock mechanics) from the University of Tehran, an MS in mineral engineering from the University of Minnesota, and a PhD in mining engineering (rock mechanics) from the University of Missouri-Rolla.
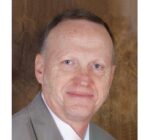
George E. King, SPE, is a consultant with Viking Engineering on well completions, interventions, and well failures. He has 48 years of oilfield experience starting with Amoco and is a former distinguished engineering adviser at Apache Corp. He is a registered professional engineer in multiple states. King earned a degree in chemistry from Oklahoma State University and in chemical engineering and petroleum engineering from the University of Tulsa, where he was an adjunct professor. He has written more than 60 technical papers and was awarded the 2004 SPE Production and Operations Award and the 2012 Engineer of the Year award from the Houston Region of the Texas Society of Professional Engineers.