The Burgan reservoir in Kuwait has the potential for premature water breakthrough, leaving behind bypassed zones of oil. The placement and completion of horizontal wells in such reservoirs is a challenge necessitating a collaborative approach from petroleum geoscience, reservoir engineering, and petroleum engineering. This paper describes placement of horizontal wells in this kind of heterogeneous reservoir through an integrated approach.
Introduction
The Minagish field was discovered in 1959 and is located in the southwestern part of Kuwait. It contains several reservoir intervals in its stratigraphic column, varying from Early Jurassic to Late Cretaceous. The field is situated 12 km northwest from the West Umm Gudair field (Fig. 1). The field has been penetrated by more than 180 wells. The field structure of the Burgan formation is a closed elongated asymmetrical anticline oriented in a north/south direction. The top of the Burgan structure is located at approximately 5,500-ft true vertical depth (TVD) minus the elevation above mean sea level of the depth reference point of the well.
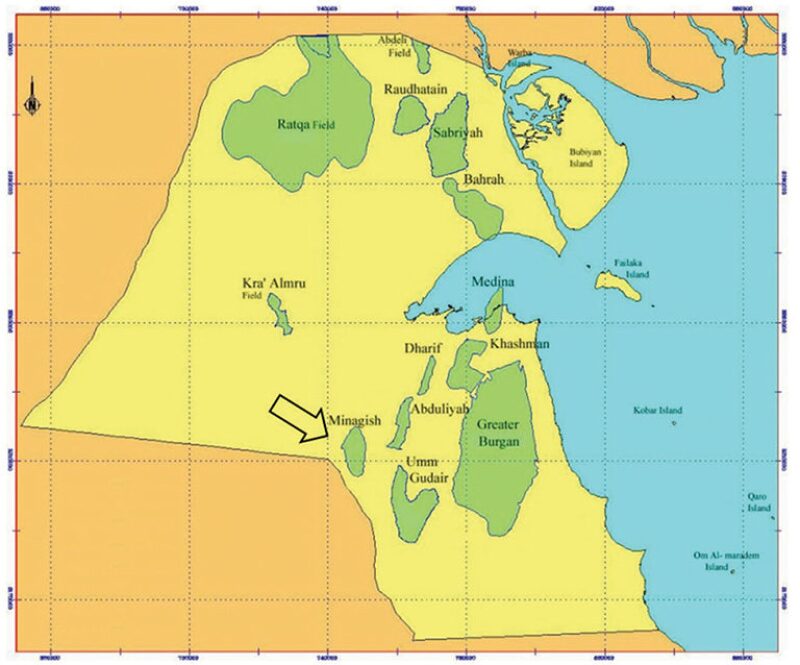
Regional Geology. The Burgan formation consists of a lower braided river system with stacked sand bodies. The sediment ranges from fine to medium grain sizes, and the porosities are high enough for lateral migration. On top of the upper Burgan channels, the sediments are generally finer but still retain a fluvial character. The amount of shale is higher. The marsh and tidal sediments, which consist of fine sands, silty sands, and sandy silts, seem to be of lower connectivity for vertical migration. The porosity is relatively low (between 15 and 18%), and the minimum of the measured permeability is close to 1 md for these layers. It seems that these shaly sediments can act as permeability barriers for vertical migration, but oil is observed in all channels and has been able to reach the reservoirs. This complex channel geometry makes these reservoirs the most challenging clastic reservoirs in the Minagish field.
The Burgan reservoir is informally divided into upper and lower sections. Lower Burgan sands are more extensive and blocky in nature, with few variations in their properties. The lower Burgan reservoir section lies above the oil/water contact (OWC) and is of significance from a hydrocarbon-bearing perspective. Upper Burgan sands are mainly in the form of channel sands, ranging in thickness from a few feet to nearly 45 feet and having extensive lateral facies variation. The lower part of the Burgan reservoir has active bottomwater drive, whereas the upper part of the reservoir has an edgewater-drive system. The reservoir contains high-permeability sands on the order of a few darcies associated with active faults and has highly viscous reservoir fluid with a viscosity of approximately 40 cp at reservoir conditions. This heterogeneous nature of the reservoir accelerates water movement inside the reservoir and results in premature water breakthrough in existing vertical and horizontal wells, in spite of maintaining high standoff from the OWC.
Scope of the Work. The current work is an integrated approach combining X-ray fluorescence (XRF), logging-while-drilling (LWD) data, and petrophysical interpretations in real time to geosteer and place horizontal wells successfully in the zones of interest with the maximum possible drainage area. After successful implementation of the workflow in the lower lateral (LAT-0) of the first well (Well A) placed in the lower Burgan, the workflow was used to geosteer and place the upper lateral (LAT-1) of Well A in the upper Burgan and both laterals of Well B (in the lower and upper Burgan, respectively). This paper uses the results of LAT-0 of Well A in general except where it is necessary to show some important features from other laterals.
Prejob Modeling
Prejob modeling is an important aspect of geosteering because it not only enables understanding of reservoir challenges but also helps to develop possible solutions to mitigate them. In the current work, the prejob modeling consisted of (a) a geosteering model based on offset-well logs, geological information, and geophysical information, and (b) a geochemical model based on XRF analysis of core chips from offset wells.
Geosteering Prejob Model. A 3D geosteering model was built with offset-well-log data and structural grid data of the lower and upper Burgan formations, to predict log responses through the desired high-angle section.
The main challenges from the upper and lower targeted zones are as follows:
- Faults encountered in the lateral sections have a remarkable amount of throws.
- Significant dip changes associated with faults or with no association with faults.
- Lateral facies variations.
Generally, there are three methods used for well placement, depending on the well complexity, the objectives, and the geological markers’ availability for lateral correlation. Because of known uncertainties in this field, the first method of geosteering based on conventional LWD tools was found to be inappropriate. The second geosteering method, based on borehole images, was not considered a primary tool for geosteering strategy but was not excluded because of a potential application (fractures and faults identification). The third geosteering method, based on deep directional measurements, was chosen and used as the primary tool for geosteering strategy; the few markers available in the targeted upper and lower Burgan sands were used as boundary references in order to place the wellbore accordingly within the targets (Fig. 2). The main challenges were to identify how far the boundaries could be seen in these particular zones and to establish the geosteering strategy for drilling the lateral sections.
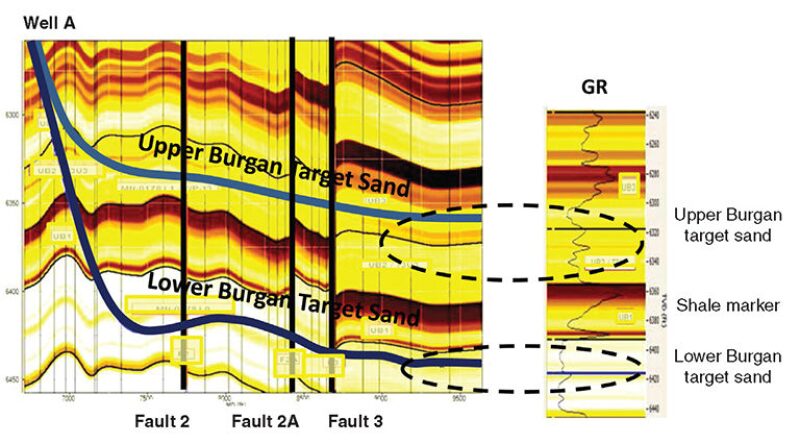
Geochemical Prejob Model. Pilot Study. Before building the geochemical model on the basis of the offset well (please see the complete paper for details about the offset-well study), a pilot geochemical study was conducted on three Wara-Burgan wells. The high-resolution geochemical analysis defined 10 chemostratigraphic packages and 15 chemostratigraphic units for the Wara-Burgan reservoir of the Minagish field.
The pilot geochemical study of the upper Burgan reservoir shows an increase in heavy-mineral contents, particularly in the middle part of the main clean-sand channel, and then an increase in dolomite content followed by maximum elevated iron values in the lower part. This is followed by increasing uranium/thorium and potassium/thorium ratios, indicating dirty-sand and shaly sections at the lowest part (Fig. 3).
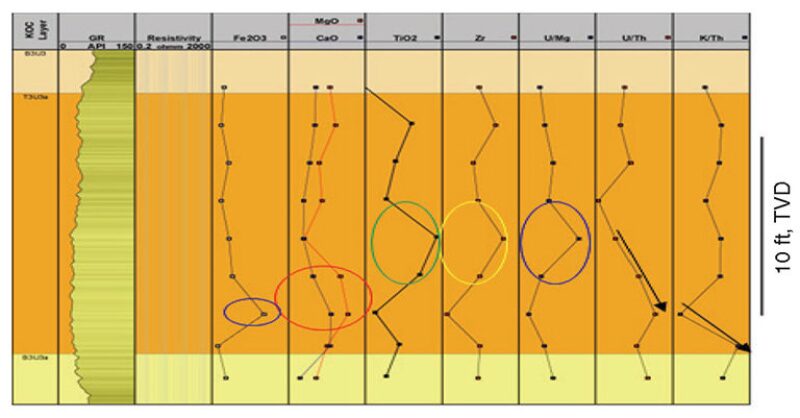
Well-Location Optimization
The well locations for smart multilateral wells are optimized by integrating data from multiple disciplines, from the macroscale (seismic) to the microscale (petrography). Furthermore, data from seismic, geology, petrophysics, reservoir engineering, and well surveillance were incorporated into the predrill characterization program. The lower lateral, LAT‑0, is placed in the lower Burgan, consisting of a braided river system with stacked sand bodies. The sediment includes fine‑, medium-, and coarse-grain sizes, with porosity ranging from 20 to 30% and permeability values on the order of a few darcies. The bottom part of the massive sand bodies is directly connected to the bottom aquifer.
The upper lateral, LAT-1, of the smart multilateral well is targeted in the upper Burgan, consisting of silt to medium sands. The porosity is relatively low (between 15 and 18%), and the permeability values are on the order of hundreds of millidarcies because the reservoir still retains a fluvial-sand character. There are shaly sediments between the lower and upper Burgan regions, which can act as a permeability barrier or as a baffle for vertical migration of fluids. This complex channel geometry makes these reservoirs extremely challenging for implementation of smart multilateral wells. The well trajectories were optimized to encounter the best reservoir sections by minimizing the exposure to fault networks as interpreted from high-resolution seismic.
Real-Time Geosteering Operations
Advanced multilateral-well drilling/completions requires the application of innovative technologies while drilling to place the well in “sweet spots” by managing geological uncertainties. The smart multilateral wells were drilled by integrating advanced high-resolution chemostratigraphy (XRF) and advanced gas analysis along with real-time geosteering formation characterization and seismic-data interpretation. The prejob chemostratigraphic analyses of offset-well data and modeling have provided reference indications for geochemical steering to characterize various facies in heterogeneous formations of the Burgan reservoir. The high-resolution 3D-seismic-data interpretation has allowed refinement of the geological model in terms of faults and reservoir boundaries. The real-time geosteering is performed by use of advanced and innovative technologies, including high-resolution XRF geochemical analysis, to identify geochemical “proxies” and allow geochemical steering. The optimum use of geochemical models was a major asset while steering the multilateral well in the complex structure of Burgan. In addition, azimuthal deep resistivity as a tool for distance-to--boundary measurements, at-bit measurements, and petrophysical interpretation has been used in real time to correct well positioning and locate faulted areas. For a detailed discussion of the XRF-aided chemosteering results and azimuthal resistivity measurements, please see the complete paper.
Real-Time Petrophysical Evaluation. In the present work, real-time log-based petrophysical evaluation complemented with XRF analysis and mud-logging data was used to better geosteer the wellbore in the zone of interest and maximize the drainage area. In the complete paper, details are presented from an integrated interpretation based on the real-time data set for the LAT-0 section of Well A. A similar approach was adopted for the LAT-1 section of Well A and for both sections of Well B.
Identification of Faults/Fractures. An azimuthal lithodensity image was interpreted while drilling, not only to understand the formation dip but also, most importantly, to identify clusters of fractures/faults; this process was complemented with XRF analysis. Fig. 4 shows four fault zones identified through the LAT-0 section of Well A; these were quite evident from XRF analysis.
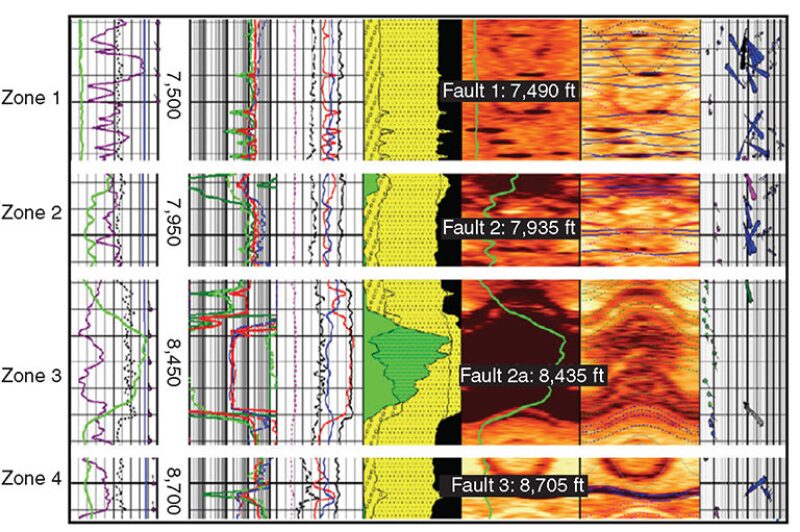
Nature of Fractures/Faults. With a limited data set, it is difficult to interpret whether the fractures are open or healed. Nonetheless, an attempt was made to understand the nature of the fractures through crossplots, which indicate that 92% of the drilled section is clean sandstone with excellent reservoir quality. Meanwhile, saturation analysis performed while drilling (by use of Archie’s empirical correlation) for the entire horizontal section indicates a very low water-saturation value across the entire interval. This, in turn, implies no water along the fractured zones, supporting the previous interpretation performed on the basis of total- and sonic-porosity crossplots that indicate that the fractures are closed or healed. Both resistivity and Pickett-plot analysis show no water across fractures/faulted zones, which is in agreement with XRF-data analysis.
Rock-Quality Indexing. Quick volumetric analysis was conducted and a few crossplots were created to understand the rock quality over the drilled interval and plan for the completion design. The rock type and quality interpreted from log data were validated further through XRF analysis and cuttings description. The rock quality, along with other LWD data, was used to geosteer the well successfully. For a detailed discussion of these findings, please see the complete paper.
This article, written by JPT Technology Editor Chris Carpenter, contains highlights of paper OTC 24002, “Innovative Geosteering Technology Used in Drilling Smart Multilateral Wells, Kuwait,” by T. El Gezeery, A. Aziz Ismael, K. Al-Anezi, G.S. Padhy, Fawaz Al Saqran, A. Abdul-Latif, and J. Silambuchelvan, Kuwait Oil Company, and J. Estarabadi and G. Ferroni, Geolog International, prepared for the 2013 Offshore Technology Conference, Houston, 6–9 May. The paper has not been peer reviewed. Copyright 2013 Offshore Technology Conference. Reproduced by permission.