When asked to describe Quaise Energy, Chief Financial Officer Kevin Bonebrake said, “At its simplest, we will manufacture steam.”
Their goal is to produce steam that is hot enough to power an electric generator built to run on fossil fuel. They plan to do that by injecting water down 10–20 km to where the rock temperature is 400 to 500°C.
The motivation for drilling wells into extremely hard basement rock is to make geothermal electric power affordable by producing steam that could be used to repower older plants connected to power grids serving major markets.
In the past, geothermal power has been little used because there are few places in the world with the right combination of the hot rock, water, and permeability required to deliver high‑quality steam.
Hot, dry rock is commonly available, but producing the supercritical steam needed requires drilling to depths of 10 to 20 km, depending on the temperature gradient in that part of the US. At that depth, the driller will be faced with rocks such as granite and basalt. The rock is capable of both producing superheated steam and destroying the microchips and seals required for directional drilling.
Quaise plans to drill ultradeep holes by generating high-powered microwaves at the drilling site. The microwaves will be transmitted downhole to make hole by melting and vaporizing the basement rock. The only downhole equipment is a long tube to guide the waves to their target.
It is one of those ideas where the first reaction most people have is “that sounds like science fiction,” which speaks to both the problem and the proposed solution.
“You have to think differently to deal with that type of temperature,” said Henry Phan, vice president of engineering at Quaise, whose job is to turn this invention into engineering reality.
Where It’s Hot
The high barriers to drilling wells 10 to 20 km deep (33,000 to 66,000 ft) beg the question, isn’t there an alternative?
A simpler, faster, and cheaper option would be to use proven drilling methods to reach not‑so‑deep levels where the steam produced is not as hot. Phan described it as “colder, lower‑pressure, wetter steam.”
That sort of geothermal energy can be useful for purposes such as providing heat for desalination or energy for central cooling systems, according to an analysis of Oman’s efforts to tap the energy of hot-water zones beneath oil fields. These zones were not hot enough to generate electric power.
There is talk of using steam from zones that are not as deep, but Quaise’s analysis concluded that the large investment required to build power plants capable of using that steam for electric generation would make the output uneconomically costly.
Quaise argues that finding a way to drill extreme wells is worth the cost and technical risks that come with new technology and drilling into little-known formations because it would save the cost of building a power plant. Its goal is to supply older fossil-fueled plants whose owners would be eager to convert to geothermal steam because of the staggering cost of complying with environmental regulations.
Based on Quaise’s cost analysis, the lifetime cost per kWh of converted power plants running on its geothermal steam would be competitive with the cost of coal and gas and a fraction of the cost of lower-temperature geothermal steam.
That assumes they can develop a method to dependably drill a large-diameter wellbore (around 8.5 in.) down that far for a price that is not a deal killer.
To make this a reality, they plan to use a mix of conventional and microwave drilling methods. They would use rotary drilling, which is faster and cheaper, until the combination of extreme heat, hard rock, and long tripping times make it the costly option.
“We will drill as deep as you can using the cheapest, most economical way to go,” Phan said. While recent testing has shown it is possible to drill faster and longer using a PDC bit in granite, “at some point the economics (of rotary drilling) do not work and we will then swap out,” he said.
Microwave drilling is not as fast, but the simplicity of the downhole equipment—a long, straight tube called a wave guide which ends at a safe distance to the well bottom during drilling—is expected to limit nonproductive time compared to rotary drilling in granite, which rapidly wears out drill bits. At 30,000 ft, hardware repairs are time‑consuming.
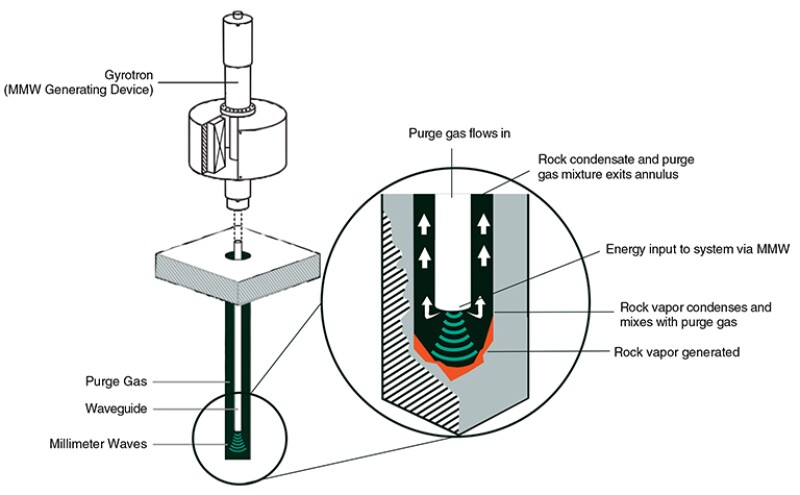
Microwave energy would be generated on the surface with a unit called a gyrotron powered by portable diesel generators or grid power.
Drillers would likely describe a wave guide, which delivers the microwave energy downhole with a minimum of energy lost, as looking like a skinny drillpipe.
Two of Quaise’s major investors also have deep connections to the oil business. They are the big drilling contractor, Nabors Industries, and equipment supplier, TechEnergy Ventures, which is part of Techint Group. Tenaris, a major maker of oilfield tubular, is part of Techint. If microwave drilling ever takes off, wave guides and automated ultradeep-drilling equipment might be a way for them to profit from the energy transition.
Proven for Plasma
The basic components used for microwave drilling are proven—just not for drilling wells.
The gyrotron has been an essential tool in the decades-long pursuit of an efficient way to generate power by fusing hydrogen atoms to create helium and energy. Its role has been creating the ultrahigh-temperature plasma that is contained within a magnetic field.
The method Quaise is trying to commercialize was developed by Paul Woskov, a research engineer at the Massachusetts Institute of Technology’s (MIT) Plasma Science and Fusion Center. At the time, he was looking for an application of high-energy microwave technology, more likely to be used in his lifetime than fusion energy.
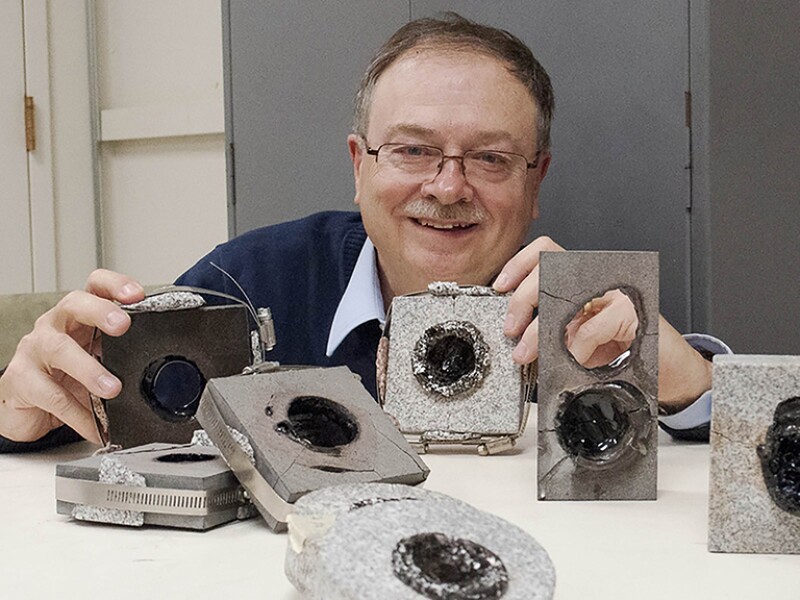
“I thought why not direct these high-power beams, instead of into fusion plasma, down into rock and vaporize the hole?,” said Woskov in an online MIT story.
Previous research on microwave drilling used lower-energy microwaves to stress the rock, allowing a drill to grind it off faster with less wear.
The method Woskov envisioned would be conceptually like using a laser for cutting, but the larger millimeter-size microwaves would be a better match for creating larger-sized holes.
Hard rock samples used for testing showed each with a round hole with a dark perimeter with the texture of hardened lava or rock, depending on the rock. If rock is uniformly fine‑grained, the extreme heat can vitrify it, creating a glass wall.
Quaise was co-founded by Carlos Araque, its chief executive officer, a former Schlumberger engineer with product development experience. He learned about the microwave drilling method when he was the technical director for MIT’s venture capital firm, The Engine.
The company holds the exclusive rights to MIT’s patent on using microwaves for drilling and has applied for and been granted additional patents based on what it has learned since it started in 2018.
Quaise’s goal is to drill the wells required to power a pilot power plant by 2026 and deliver a commercial system by 2028. For now, the 28-member engineering team, located near Houston, in Boston, and Cambridge, UK, is working on drilling longer holes in the lab and preparing for field testing in the next couple years.
This year, their goal is to drill a 100/1 hole—that is, a 100-in.-long, 1-in.-diameter hole, or ten times their previous best. At the same time, they are designing the more powerful, durable equipment needed to drill larger, longer holes in the field.
To do so they have ordered a 1-MWh gyrotron that will allow them to scale up on a site where the drilling rig that drilled the upper hole would handle the wave guide as the well went deeper.
“We are designing the rig to take all this equipment they have been using in fusion experiments and ruggedizing it and making it so it can be used with a typical land rig,” Bonebrake said.
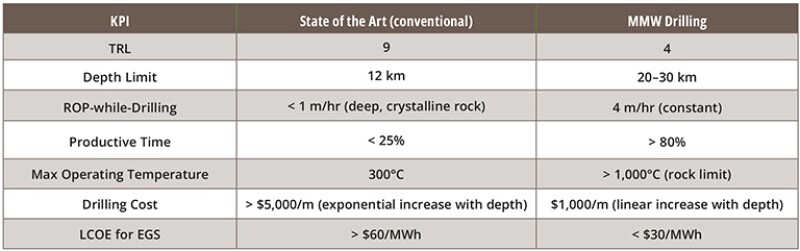
What’s So Hard?
Compared to rotary drilling, making hole with microwaves looks as simple as shooting a ray gun.
The only downhole equipment is the wave guide. The tube needed for a wave guide is considerably lighter than that used for drilling because the tubing diameter is narrower and not nearly as thick. This is possible because the weight on bit and torque are not factors in microwave drilling performance.
There are no moving parts downhole, but there are a lot of proprietary design elements in the wave guide, from how it widens at the exit point to stimulate a consistently sized area to modifications inside the tube to limit energy loss.
Maintaining a straight well path is critical because bends can interfere with an energy beam that travels in a straight line.
That would require tight management of the wellbore quality problems that are commonly found in oil wells, where well path surveys based on downhole measurements while drilling include an area called the “cone of uncertainty.”
Concerns about interference also affect plans for how to manage well cleaning. When switching to microwave drilling, they plan to use a high‑pressure stream of gas to clear the drilling debris, which Bonebrake described as “fine vaporized particulates.”
Two options in play are nitrogen and air. Air is cheaper but contains some water.
The microwave beam can deal with small amounts of water encountered while drilling; Phan said the “microwaves can vaporize it.” But that would not work if they drilled into a large water‑filled fault with high permeability. Such hazards are “unlikely to occur in deep basement rock,” Bonebrake said.
A professor whose work has focused on the properties of formations at these depths said hot, dry rock is not totally dry.
“There are indications of fluids throughout the brittle crust (typically the upper 15 to 20 km),” said Mark Zoback, a geophysics professor at Stanford University. He added that the “water is concentrated in fractures and faults, but the matrix is also saturated, although the porosity and permeability are quite low.”
Zoback coauthored a paper in Geology (2000) that cited several studies measuring the permeability in basement rock. It reported large differences in the permeability observed in small rock samples vs. wider-area measures based on pressure testing. It explained that gap by pointing out that the large areas included fractures and faults, some isolated and some connected to larger networks of fissures.
“The high permeability, three to four orders of magnitude higher than that measured on core samples, appears to be maintained by critically stressed faults and greatly facilitates fluid movement through the brittle crust,” the authors wrote.
While describing the data sources, the authors showed how few research wells have been drilled at those depths. While there have been some extremely deep oil wells drilled, they were not in granite because hydrocarbons are so unlikely to be there.
Quaise’s goal is to drill well past the deepest of the research wells mentioned in the paper—the Kola Superdeep Borehole in Russia which was 12.2 km deep. All the rest were less than 10 km deep.
If they hit a water-filled fault in extremely deep rock while microwave drilling, Phan said their first option would be to seal the wellbore “as we are drilling and keep the water behind the vitrified wall. If the volume of water is significantly more than we can seal, then we can turn this into a traditional hydrothermal well.”
Truly hot, dry rock presents its own challenges. Generating steam will require finding ways to create flow paths from water-injection wells to steam producers. Those fractures will need to be constricted enough to ensure the water is exposed long enough to the rock to become superheated. Volume also matters because they need to limit the number of costly wells required.
On the plus side, the paper on deep permeability said there are natural fractures to help create such networks. Bonebrake and Phan said the company is in touch with multiple companies working on the problem, which is also the focus of a federally funded research project at the US Department of Energy’s Utah FORGE facility.
“This is all stuff that needs to be proven out,” Phan said.
For Further Reading
How Faulting Keeps the Crust Strong by John Townend and Mark D. Zoback. Geology (2000) 28(5): 399–402.