Proper lateral and vertical well spacing is critical for efficient development of unconventional reservoirs. Much research has focused on lateral well spacing but little on vertical spacing, which is challenging for stacked-bench plays such as the Permian Basin. Following a previous, successful single-well study in paper SPE 189855, the authors have performed a seven-well case study in which the latest complex fracture modeling and reservoir-simulation technologies have been applied. This synopsis will concentrate on the methodology behind the study; the reader is encouraged to view the complete paper for specific comparisons of completion designs.
Introduction
Complex-fracture-modeling tools are used frequently to study well spacing.
×
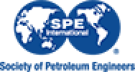
Continue Reading with SPE Membership
SPE Members: Please sign in at the top of the page for access to this member-exclusive content. If you are not a member and you find JPT content valuable, we encourage you to become a part of the SPE member community to gain full access.