The complete paper presents a new three-phase relative permeability model for use in chemical-flooding simulators. A model that has been widely used in chemical-flooding simulators for decades has numerical discontinuities that are not physical in nature and that can lead to oscillations in the numerical simulations. The proposed model is simpler, has fewer parameters, and requires fewer experimental data to determine the relative permeability parameters compared with the original model.
Background
Two- and three-phase relative permeability measurements at low interfacial tension (IFT) have been published previously, and microemulsion relative permeability models have been proposed in the literature as well. But none of these can model the microemulsion phase across different phase-behavior environments, from oil-in-water, to the middle phase, to water-in-oil emulsions.
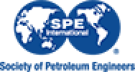