Accurate monitoring of the ground deformation of a field under cyclic steam stimulation (CSS) can be used to calibrate predictive models, show the effectiveness of steam injection, and demonstrate the effect of the recovery operation on the surface elevation. Interferometric synthetic aperture radar (InSAR) uses radar returns from the ground to calculate highly precise estimates of the ground change. The authors discuss a new way of extracting deformation information from radar imagery, contributing to improved accuracy of InSAR surface-elevation monitoring.
Introduction
The challenge in the Canadian oil sands is to achieve robust measurements with limited infrastructure. Essentially, the problem is to develop robust measurements of the ground movement without reliance on buildings, pipelines, or even installed targets [such as corner reflectors (CRs)]. The case study described here shows exceptional results for monitoring of regions without traditional point targets.
The operations and mechanism of thermal recovery of steam-assisted gravity drainage (SAGD) and CSS differ.
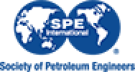