This study focuses on recent experience in Saudi Arabia with crude-oil compositional analyses during pumpout with a wireline formation tester (WFT). It summarizes experience with the in-situ measurement of methane, ethane, propane, saturates, aromatics, and gas/oil ratio (GOR) on the basis of multivariate optical computing (MOC) conducted at more than 200 pumpout stations in a total of 37 wells drilled with a variety of inclinations, bit sizes, and drilling fluids in several oil and gas fields.
Introduction
In reservoir-fluid characterization performed in the laboratory conventionally, samples of representative formation fluids are analyzed to determine bulk fluid properties, fluid-phase behavior, and chemical properties. Exploration and evaluation wells are often drilled exclusively for fluid-analysis purposes for which the only way to analyze or capture formation fluids is a downhole pumpout WFT (PWFT). Capturing high-quality reservoir samples is one of the most important objectives in any PWFT job.
The keys to ensure fluid cleanup during pumpout are (1) a set of downhole sensors measuring the pumped fluids and (2) the unambiguous contrast between the drilling-mud filtrate and reservoir fluids as measured by at least one of these sensors. Avoiding such fluid ambiguity is a challenge in many situations. Optical sensors respond with high sensitivity to chemical compositions of fluids.
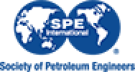