Triazines are commonly used as hydrogen sulfide (H2S) scavengers. However, stress corrosion cracking (SCC) has been reported recently when using certain triazines, making it necessary to understand the failure mechanism to control this phenomenon. In the complete paper, the authors seek to understand the effect of triazine on the electrochemical response of a carbon-steel surface in a mixed-gas system.
Introduction
One of the more frequently used H2S scavenger triazines is hexahydro-1,3,5-tris(hydroxyethyl)-s-triazine, or monoethanol amine (MEA)-triazine. It is a yellow, viscous liquid that can be injected into gas streams or used in a contactor tower.
×
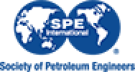
Continue Reading with SPE Membership
SPE Members: Please sign in at the top of the page for access to this member-exclusive content. If you are not a member and you find JPT content valuable, we encourage you to become a part of the SPE member community to gain full access.