Multiple challenges related to liquid loading have been observed during flowback after hydraulic fracturing and during the production phase. These challenges are further aggravated by high-inclination angles found in deviated wellbores. The complete paper describes an experimental study performed to investigate the onset of liquid loading in a 6-in. production casing at various inclination angles.
×
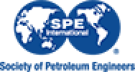
Continue Reading with SPE Membership
SPE Members: Please sign in at the top of the page for access to this member-exclusive content. If you are not a member and you find JPT content valuable, we encourage you to become a part of the SPE member community to gain full access.