The complete paper proposes an azimuthal plane-wave-destruction (AzPWD) seismic-diffraction-imaging work flow to efficiently emphasize small-scale features associated with subsurface discontinuities such as faults, channel edges, and fracture swarms and to determine their orientation by properly accounting for edge-diffraction phenomena. The work flow is applied to characterize an unconventional tight-gas-sand reservoir in the Cooper Basin in Western Australia. Extracted orientations of edges provide valuable additional information, which can be used by the interpreter to locate finer-scale features and distinguish them from noise.
Introduction
Unconventional reservoirs may exhibit high structural variability, which is difficult to characterize with a discrete wells network. 3D reflection seismology allows the extraction of additional information about the subsurface with significantly denser spatial sampling intervals.
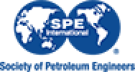