ExxonMobil proposed this week to build what would become the world’s biggest carbon capture and sequestration (CCS) project in the Houston area. The supermajor’s plan is to capture the CO2 from the petrochemical and industrial facilities that line the Houston Ship Channel and then transport it via a subsea pipeline to an offshore reservoir.
The realization of this megaproject will depend on a number of other private and public stakeholders to help fund it and to create new policies that will facilitate the growth of the CCS sector in the US. It will also require the skills of petrotechnicals who will be challenged with de-risking the region's formations to find those that are truly suited to absorb the vast volumes of CO2.
The company estimates the Houston-based CCS hub could reach an annual capacity of 50 million metric tons (Mt) by 2030, and 100 million Mt tons by 2040; a figure that represents a sevenfold increase of total capacity in the US today.
On a global basis, ExxonMobil already sequesters about 9 million Mt of CO2 each year. About 7.4 Mt of that figure is injected into the subsurface at the company's Shute Creek facility in Wyoming, currently the world's biggest CCS project.
With an estimated price tag of at least $100 billion for its next big leap, ExxonMobil is asking for government support at all levels—federal, state, and local—to create within Houston a “CCS Innovation Zone.” The scheme would be similar to other economic zones that governments have established around the world to promote the domestic development of strategic sectors.
“Houston has two features that make it an ideal site for CCS: It has many large industrial emission sources, and it’s located near geologic formations in the Gulf of Mexico that could store large amounts of CO2 safely, securely and permanently,” Joe Blommaert, the president of ExxonMobil’s Low Carbon Solutions division, said in a statement.
Blommaert, who was appointed to head the newly created business unit in February, also reiterated the company’s support for a carbon price which he said “will play an important part by providing the needed clarity and stability required to drive investment.”
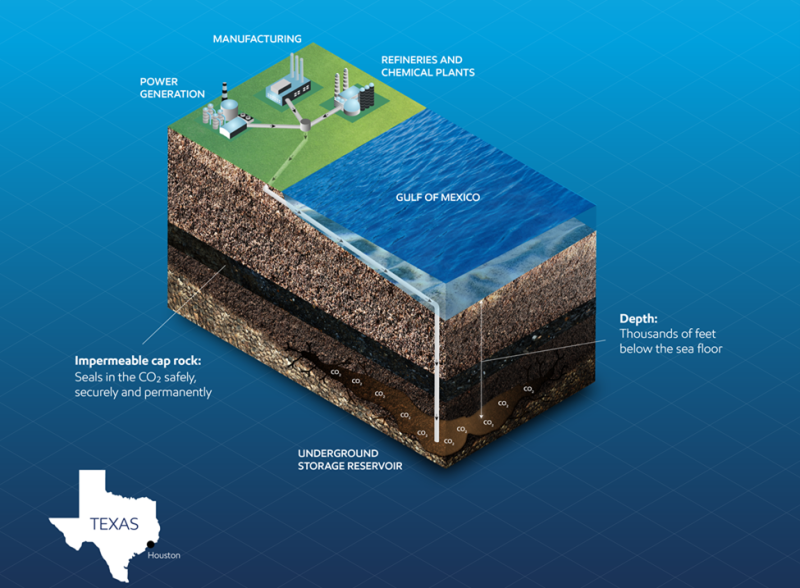
Where Will It All Go?
The announcement from ExxonMobil cited figures from the US Department of Energy that estimate the US Gulf Coast has a CO2 storage potential of around 500 billion Mt. This is equal to more than a century’s worth of emissions from the US industrial and power generation base.
A 2020 peer-reviewed paper (SPE 196723) by researchers from The University of Texas at Austin (UT) Energy Institute also highlighted that the region holds “favorable formation properties” for permanent sequestration. Their research, which was supported and guided by ExxonMobil, offers one potential target for the supermajor’s CCS project: deltaic saline aquifers.
These aquifers were formed millions of years ago as rivers drained into slower-moving seas and oceans. Some deltaic formations accumulated large amounts of hydrocarbons, which has made them a favorite target of oil explorers the world over.
But just as it is important to find the right drilling location within these large structures when they are laden with oil, so too is it to understand what makes one deltaic aquifer more ideal for CCS than another.
To help operators better screen candidates in the future, the UT researchers developed what they describe as a "unique and extremely well-characterized" geologic model and a simulation workflow. Their model recreates a deltaic formation in the Gulf of Mexico that ranges in depth from 1000 m to 2500 m.
A central focus of the paper is on the way different rock types and their architecture are likely to affect the way the injected CO2 migrates inside the geologic storage sites.
The analysis suggests that shale layers are a highly effective cap, or flow barriers, for the CO2. The simulations showed that in shale-capped scenarios, CO2 retention was as high as 100%. As the CO2 hits the caprock, it stops flowing vertically and begins migrating laterally until it finds another vertical pathway. In an open boundary scenario, e.g., a sandstone lacking a structural barrier, the storage rate falls to 6.6%.
Injection rates are another major factor for operators to consider when it comes to geologic candidate selection. In a closed scenario with a homogenous sandstone, the simulations show how bottomhole pressures are likely to build up quicker than in open formations, resulting in a lower ceiling for injection rates. And because of rock homogeneity, this setting also results in less areal propagation of the CO2 as it preferentially flows to the top of the formation.
A more ideal result might be obtained if the target rocks are heterogenous, with varying degrees of capillary pressure and saturation levels that allows for more spatial distribution of the gas. In this case, the CO2 plume that has been created by the injection process may remain relatively static in shape for up to 1,000 years after injection has ceased.
What will change though is the nature of the CO2. It will transition from free gas, or mobile gas, to an immobile phase where it can be trapped between rock layers, or in isolated blobs within the rock’s network of tiny pores. The UT researchers noted that a smaller but still significant volume is likely to dissolve into the aquifer’s brine.
For Further Reading
SPE 199975 Carbon Dioxide Storage in Deltaic Saline Aquifers: Invasion Percolation and Compositional Simulation by S. Tavassoli, P. Krishnamurthy, E. Beckham, T. Meckel, K. Sepehrnoori, the University of Texas at Austin.