A nearly 70-year-old equation has become the cornerstone of a newly emerged approach to reservoir diagnostics and well appraisal at two of the largest shale companies in the US.
With many North American tight-oil producers overseeing low-price-driven shut-ins of horizontal wells, this development has become increasingly relevant. As wellhead prices rise and the shut-in population is brought back into the fold, a window of opportunity exists for operators to run late-life interference tests on wells they otherwise likely would not have planned for.
One challenge though will be in selecting the ideal testing model.
Outside of the traditional approaches, the Chow Pressure Group (CPG) has been proposed as a promising candidate and one that is advantageous to all but the most price-sensitive and resource-strapped operators of shut-in assets.
Described by its adopters as a simple equation, it comes from the not-too-distant world of hydrology where it was introduced in the 1950s as a groundbreaking method to analyze the flow behavior from freshwater aquifers.
In 1987, the oil and gas industry would be introduced to CPG by one of its own academic luminaries: Erdal Ozkan, a professor of petroleum engineering at the Colorado School of Mines, an SPE technical director, and the author or coauthor of more than 100 technical papers—including SPE 16378.
In that paper, he and two other researchers showed that CPG responses are identical to pressure responses observed during a key production phase called the pseudoradial flow period. Additionally, they demonstrated how this CPG response could be plotted on a straight line when a flow regime exhibits something known as power-law behavior.
Some 30 years later, petroleum engineers at Irving, Texas-based Pioneer Natural Resources and software firm Kappa Engineering published the first of two papers (SPE 187180) that brought this work back into the fore. Using the CPG equation, they were the first to prove that the power-law behavior (and its nonstandard slope) was the norm for the majority of the operator’s wells in the Wolfcamp Shale—the most-targeted formation in the Permian Basin.
“In reality, it’s very simple. It’s the pressure change with time over the corresponding derivative slope,” said Wei-Chun Chu in offering a concise explanation of the formula of focus:
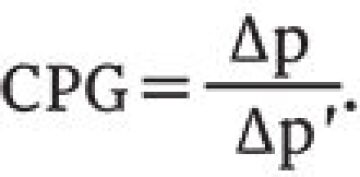
Chu is the lead author on the two papers which outline how the CPG was integrated into the well interference test program at Pioneer where he retired from a year ago. The equation is now a highly influential tool that Pioneer uses to guide major decisions on well spacing, completions volumes, and its grading of the results of stacked and staggered well patterns.
The veteran reservoir engineer points out that despite its simplicity, adopting this new approach requires discipline. “You have to do this very systematically,” Chu cautioned. “People will have to do their homework to get everything organized in order to understand the bigger picture.” For those who do their due diligence, the reward is a quick and accurate way to estimate the magnitude of pressure interference (MPI) between offset wells.
In one of its published case studies, Pioneer noted that as a result of using the CPG method it decided to tighten up fracture cluster spacing in a development area. The correlating outcome included lower MPI values, increased productivity, and better overall economics (results will vary depending on both geologic and completion design factors).
Pioneer’s experience has since been shared by others—the fastest follower being Devon Energy.
“Within Devon, we’ve found interference testing to be very valuable, and now is a perfect time and opportunity to conduct some of those tests,” said Trevor Ingle, a completions engineer at the Oklahoma City-based operator.
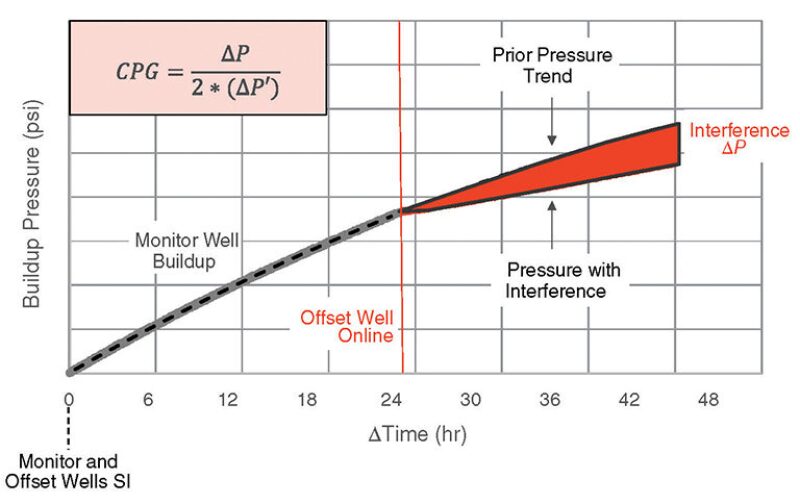
An example of the Chow Pressure Group as plotted during an interference test between offsetting wells. The approach involves running a downhole gauge in one of several wells; monitoring buildup pressures and obtaining stable conditions; systematically putting offset wells on production; and monitoring the deviation from stable conditions. Source: Trevor Ingle and Hart Greenwood, Devon Energy.
Ingle was speaking to industry peers during a virtual seminarheld in May to share various models that subsurface engineers can use to analyze shut-in wells—offering a silver lining to the painful tight-oil production curtailments that might be approaching 2 million B/D according to some analyst estimates.
Amid this backdrop, he presented examples from the more than 100 well-pair interference tests spread across about 20 drilling sections where Devon has applied the CPG technique. Many of these were performed during the flowback stage, but a significant number involved temporary shut ins of older wells.
These tests were done in every major basin in which Devon operates: Powder River Basin, STACK, Eagleford Shale, and its newest developments in the Delaware Basin.
Compared with other analysis techniques the company has used in the past, Ingle said that his team believes “this is the most robust, in terms of giving you a single value that consistently describes the magnitude of interference.”
Pioneer’s second paper, SPE 191407, published in 2018, highlights the speed of the analysis and its rewards, but in the context of a newly completed well scenario. “We can now predict with confidence the long-term well performance degradation due to well spacing by analyzing pressure interaction from only the first 2 days of production. This rapid evaluation allows for nearly immediate reaction time to improve planning of the next set of wells.”
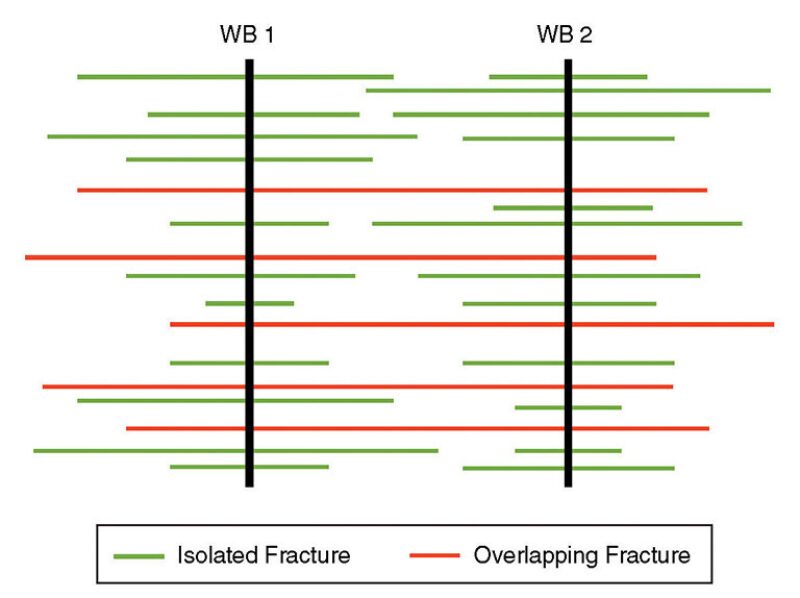
A simplistic example of what is in reality a complex network of fractures that sometimes connect, and sometimes remain in isolation. Using interference testing, one objective is to quantify this connectivity to understand development design outcomes. Source: Trevor Ingle and Hart Greenwood, Devon Energy.
New Thinking on Tight-Reservoir Flow
It is important to draw a clear distinction here between efforts to monitor frac hits, or fracture-driven interactions (FDIs), during the completion phase—though these interactions play a central role.
Instead, CPG is used during the flowback and production phases to quantify the degree of connectivity of overlapping fractures between offsetting wells that were either formed by FDIs or the activation of intersecting natural fracture networks.
This point is key to how the CPG method requires its user to depart from the widely accepted concept of normal, or linear flow, that has been applied to unconventional reservoir analysis since the onset.
Chu points out though that unconventional reservoirs are defined by complex and heterogenous environments which do not allow for normal diffusion through the tight-rock fabric. A better fit, he argues, is the anomalous diffusion concept.
Under this regime, a tight-reservoir matrix plays a secondary role to a well’s conductive fracture network, e.g., the byproduct of pressure pumping hundreds of millions of pounds of sand and water into nanodarcy source rock that is, to various degrees, pocked with natural fractures.
The two forces at play include superdiffusion and subdiffusion effects. Chu said superdiffusion implies a “preferential flow path—a very high-permeability freeway” while subdiffusion reflects the “discontinuities, obstacles, and dead ends” that delay flow into the system.
Put another way, it is all about the hydraulic fractures and the connected system in the early period of a well’s life. Later on in life, the less-permeable areas of the matrix will gradually flow.
Chu said there are many examples of where the CPG approach has been successfully utilized outside of the shale sector, including in naturally fractured conventional formations all over the world.
The foundational work supporting the anomalous flow concept was born from a group of academic experts that are cited throughout his second paper on the CPG that was republished in the February edition of the peer-reviewed SPE Reservoir Evaluation & Engineering Journal. “My job was to bridge the theorical work with the field data,” shared Chu.
From Water World to the World of Hydraulic Fracturing
In addition to the academic researchers of anomalous flow, another pair of shoulders that Chu stood upon in the pursuit of his work was its namesake—a hydrology professor at the University of Illinois, Ven Te Chow.
Chow was a world-renowned water resources engineer who in 1952 published a testing procedure to analyze the drawdown behavior of freshwater aquifers. His works are considered required reading for anyone pursuing a career in the field of hydrology.
Chu’s connection to the late expert on connectivity came while he was earning his PhD from the University of Tulsa in the early 1980s. His advisor and professor there, Raj Raghavan, would also become the advisor of Ozkan while he was earning his PhD a few years later. The two would go on to coauthor the paper that introduced the CPG equation to the oil and gas industry, which resulted in its successful use in studying horizontal wells in naturally fractured conventional reservoirs.
“But then it kind of died out,” Chu recalled. In other words, the methodology was relegated to the industry’s ever-growing archive of nonapplied academic exercises. That is until Chu said he was challenged to help Pioneer answer pressing questions around well spacing. This was in 2016 when the company made the decision to install downhole gauges in most of its new wells in the Permian.
Chu and the subsurface team got their first chance to make good on the investment in a pad of newly completed wells that had to be brought on sequentially due to produced water takeaway constraints.
“After I collected the data, I asked, how do I analyze it,” said Chu, noting that this is the moment he reached back nearly 45 years to the memory of his professor’s use of an equation conceived a generation earlier. “That’s when I tried the Chow Pressure Group and everything lined up—it was working.”
Further underscoring the tight circle that has given rise to the method, he added that helping him along with the work was the coauthor of his first paper on the topic, Chih-Cheng Chen, a reservoir engineer and software manager at Kappa, who has collaborated with Raghavan in writing several other technical papers on anomalous diffusion.
Pressure-Only Analysis
Apart from a full understanding of the mathematics and the embrace of nontraditional flow concepts, the other chief requirement to become a practitioner of this “pressure-only analysis” is the investment in bottomhole gauges which operators typically rent from oilfield service companies.
While outlining the basics of how to run an interference test based on CPG analysis, Ingle noted that at minimum the process requires the installation of one downhole memory gauge inside a well among a pad of other shut-in wells.
The second step is to wait up to 24 hours to allow the gauge to record a reliable pressure buildup signature. Assuming the testing conditions are adequate, Ingle said within this period engineers should have all they need to make a forecast of the pressure trend, after which it is safe to begin testing wells by sequentially bringing them on line. If there is connectivity between the monitor well and the wells being activated, “the pressure gauge is going to feel that.”
When this happens, the deviation— or pressure change—from the expected trend becomes the key input needed to quantify and describe the connectivity. In another reminder of how the concept of anomalous flow underpins the CPG approach, Ingle remarked that an entire pad can be tested this way in under 48 hours “because we’re dealing with high-conductivity fractures.”
The downhole hardware required to adopt the CPG method adds cost and planning time vs. a traditional production rate test. But it also enhances the method’s flexibility because, as Ingle shared, the opportunity is not lost on wells already shut in and not being monitored. “You can still run the gauges after the fact and get good data.”
Historically, the increasingly price-sensitive shale sector has largely bristled at the widespread use of downhole gauges. Pioneer and Devon stand out in this regard. Addressing the cost issue directly, Ingle said, “When you think about downhole gauges, you may think that it’s cost prohibitive—but we found it very cost effective.”
Devon uses permanent downhole gauges in certain cases, but Ingle said by using retrievable memory gauges, or what some call “dip ins,” the barrier to entry can be lowered to a point where installation and the interference test itself can be done for less than $10,000, which he added “is well worth the data we’ve collected.”
Chu also said that the critical outputs of the CPG method were most reliable when the inputs came from a bottomhole pressure gauge. Because of the complexities involved with measuring multiphase flow, the use of surface gauges “creates more headaches” for engineers, as the data would likely lead them to highly misleading interpretations.
Such guidance is not a big surprise. As reflected in their recent contributions to industry literature (including applied research separate from this example), both Pioneer and Devon have increasingly deployed bottomhole pressure gauges to enable their subsurface teams to zero-in on various aspects of fracture dynamics.
Pioneer is considered by some to be the most aggressive on this front, placing downhole sensors in most of its new wells since 2016. The company’s first paper on the CPG method came after the analysis was applied to 400 wells. The second paper reported an updated figure of 650 wells. Since then, the number has likely swelled by hundreds more.
Leveraging the Magnitude
The output from the CPG formula, the MPI, normally ranges from less than 0.5 to 1. The metric enables an operator to categorize connections that form between wells, and if continuously tracked, how these fractures maintain or lose their connectivity over time.
An MPI of less than 0.5 may imply that there is not a significant connection between a monitor and its neighbor, while an MPI of 1 is a strong indicator of a major connection. The goldilocks zone—a scenario where offsetting fracture tips overlap, but not as a result of overcapitalizing a section—might be an MPI of 0.7. However, it may be lower or higher depending on each location’s geologic responses and completion design factors. As an index is built, the target MPI becomes clearer to its trackers.
Chu pointed out though that the goal posts move along with the completions crews.
He gave an example of how the same fracture design could be applied in two different areas. In one, the completion appears to be optimal. In another, the same design results in strong FDIs with high MPI values.
“Apparently, that’s a much better-connected area, so you can widen the spacing,” he said, referencing the implication that natural fractures are likely a dominant factor in this particular area given there were no changes to the completion.
In one of Devon’s newly shared case studies, an MPI of 0 was observed on an appraisal well drilled below a set of wells drilled months earlier. With no apparent connection or pressure deviation, the conclusion was drawn that an effective geologic barrier existed between the benches.
The operator then could more safely consider a denser well-spacing plan to drive up the value of the section. It could also elect to develop the lower section until a more opportunistic period for prices arises. Other opportunities for value creation include refining assessments of a section’s true “sweet spot,” and calibrating fracture models to better test the limits of completion volumes.
“One of the really interesting observations that we found was that the more connectivity we had—or an increase in the magnitude of pressure interference—actually led to increased recovery,” said Ingle, adding that this “made a lot of physical sense” to the subsurface team.
The team’s theory, which it now says is confirmed, was that more fracture overlap meant a larger drainage system inside the tight reservoir, or one with less bypassed reserves. The finding led the team to conduct an experiment to see if wells with common spacing perform better if proppant volumes were doubled in each fracture stage.
The additional proppant did lead to larger MPI figures. Importantly, Ingle said the larger, more connected fracture systems between the wells also resulted in increased recovery factors based on several months of production data. “We didn’t stop there because this data set provided some really interesting behavior as it relates to potentially diminishing returns,” in regard to how much more proppant can be squeezed in between wells, he said.
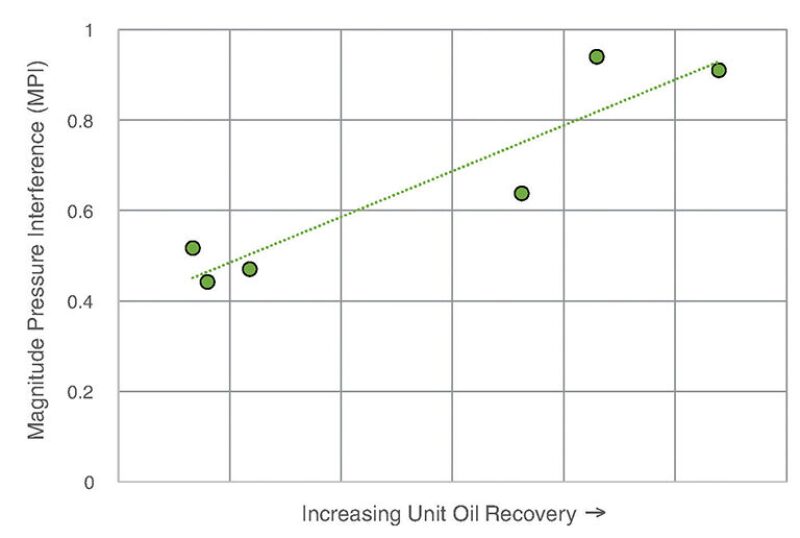
Completion volume optimization. Following interference testing on multiple units with the use of varying development strategies, this plot was formed. It shows higher-magnitude pressure-interference events increased overall unit recovery. Source: Trevor Ingle and Hart Greenwood, Devon Energy.
For Further Reading
SPE 191407 A New Technique for Quantifying Pressure Interference in Fractured Horizontal Shale Wells by Wei Chun Chu, Kyle Scott, and Ray Flumerfelt, Pioneer Natural Resources; Chih-Cheng Chen, Kappa Engineering.
SPE 187180 Rate-Transient Analysis Based on Power-Law Behavior for Permian Wells by Wei Chun Chu, N. Pandya, and Ray Flumerfelt, Pioneer Natural Resources; Chih- Cheng Chen, Kappa Engineering.
SPE 16378Horizontal Well Pressure Analysis by Erdal Ozkan and Raj Raghavan, University of Tulsa; Sada Joshi, Phillips Petroleum.