Clay minerals are an abundant component of many sedimentary formations and their presence is both a blessing and a curse.
On one hand, they yield critical insights to geologists on the dispositional environments and history of a formation. On the other hand, clay minerals are a significant contributor to formation damage.
The upstream industry defines formation damage simply as an impairment to flow caused by a reduction in permeability in the near-wellbore region. Clay enters the fray here as a leading root cause of formation damage as it absorbs water, causing it to swell. This clay swelling inevitably leads to an increase in volume that causes the surrounding rock to expand.
Another mechanism, clay dispersion, involves fine migrations of clay particles that are transported by downhole fluids.
In both scenarios, the integrity of the pore spaces is often altered and the permeability is reduced—making it more difficult to extract hydrocarbons.
This has driven the need to mitigate damage using an array of different clay stabilizers which in turn has driven the need to fully understand their makeup in order to find a match.
Meanwhile, striking the right balance between the performance of the chemistry and its sustainability is also critical to obtaining desired performance under varying conditions.
To understand the general susceptibility of clay minerals toward dispersion and swelling, a discussion of clay minerals follows.
Meet the Clay Family
Clay minerals exhibit a unique phyllosilicate structure that consists of sheet-like layers compromising varying configurations of silica tetrahedral and alumina octahedral sheets in a 1:1 or 2:1 ratio as shown in Fig. 1.
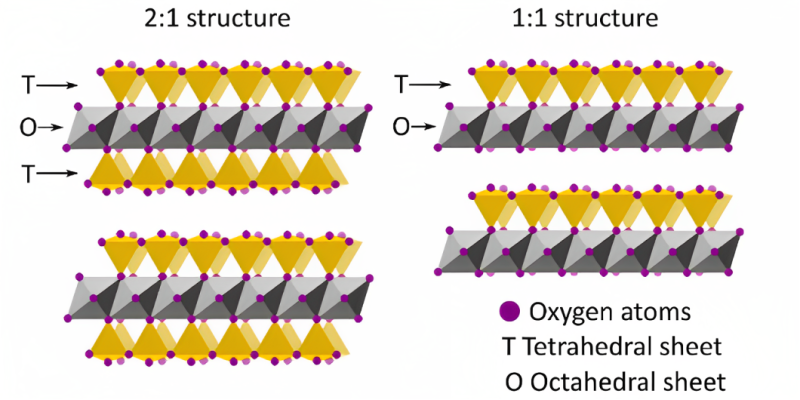
There are different types of clay minerals including montmorillonites (a member of the smectite group), kaolinites, chlorites, and illites, each of which are distinguished by a different arrangement of silica tetrahedral and alumina octahedral.
Montmorillonites consist of a layer of two silica tetrahedral sheets and one alumina octahedral sheet (2:1) which are held together by weak O-O Van der Waals forces.
The interlayer structure of montmorillonite cations such as Na+, Ca2+, Mg2+, etc. have the potential to hydrate and increase the osmotic pressure resulting in substantial swelling.
Kaolinites are known for fine migration formation damage, and they consist of one silica tetrahedral and one alumina octahedral sheet (1:1) that are bonded together by strong hydrogen bonds. The strong hydrogen bond prevents this clay type from ion exchange reducing its swelling tendencies.
Chlorites follow a similar structure to montmorillonites as they also consist of a layer of two silica tetrahedral sheets to every one alumina octahedral sheet (2:1). Unlike montmorillonites, chlorites are known to cause formation damage in the form of fine migrations rather than clay swelling.
Illites are similar to montmorillonites and chlorites as they are composed of two silica tetrahedral and one alumina octahedral (2:1) layers. In the illite clay family the cation imbedded between the layers of silica tetrahedral and one alumina octahedral is K+ which has a very comparable diameter size to the adjacent hexagonal size of the adjacent silica tetrahedron. The similar size allows the illite clay mineral to be intact, preventing any major ion substitution, and because of that illites are not known to swell but rather known for their fine migrations.
The performance of clay stabilizers is not only a factor of their structure but also a factor of their distribution. There are many distributions that clay minerals assume within a rock’s pore spaces including discrete particle distribution, pore-lining distribution, and pore-plugging distribution depicted in Fig. 2.
In a discrete particle orientation clay particles are present as discrete particles plugging the pore spaces. Meanwhile, in a pore-lining orientation clays line the pore walls of the rock.
Finally, in a pore-bridging orientation, clays bridge between different rock grains. With the different orientations in mind, clay minerals can induce formation swelling or dispersion at different locations depending on their original distribution within the pore spaces.
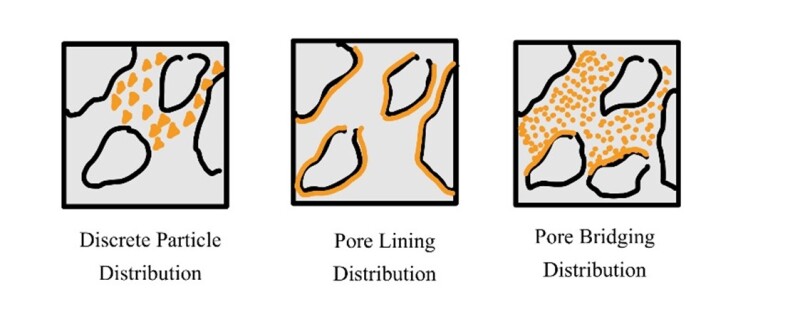
Given the challenging environment clay stabilizers must face—including the unpredictable downhole chemistry and conditions—the oil and gas industry is taking notable leaps in its development of the technology.
Newly formulated clay stabilizers have demonstrated an ability to impede formation damage and increase the longevity of producing wells. However, the precise fulcrum balance between performance and sustainability has yet to deliver the industry a one-size-fits-all formula. The absence of such a breakthrough means for the foreseeable future there will be a reliance on tailored solutions for varying downhole well conditions.
There are several types of clay stabilizers in the industry including simple inorganic salts, inorganic polymers, acids, alkalis, simple organic salts, organic polymers, cationic oligomers, and nanoparticle.
The following will shed light on each of these different types and provide an understanding of the constant tug of war between the different group of clay stabilizers given the advantages and disadvantages of each group.
Simple inorganic salts are the most common clay stabilizer group that is used in the industry and they include KCl, KNO3, NH4Cl, NH4H2PO4, CaCl2, etc. These salts prevent clay swelling by providing an influx of cations that absorb electrostatically or exchange with the original cation in the clay structure.
In general, the biggest advantage of simple inorganic salts boils down to their low cost and a low health, safety, and environmental (HSE) risk profile. Despite this, simple organic salts have their downsides. They are often needed in large concentrations, are only effective for short periods of time, and are not effective in clays that have few exchangeable ions quantified by the cation exchange capacity. The latter means if formation clays have a low cation exchange capacity, the simple inorganic salts will lose their effectiveness in preventing clay swelling and formation damage.
Simple organic salts such as tetra methyl ammonium chloride and choline chloride are also used for clay stabilization. Simple organic salts provide a temporary solution by attracting the oppositely charged clay surface and leaving the hydrophobic tail to push water molecules away. The disadvantages of these stabilizers are that they are highly toxic, provide a temporary solution, and are challenged by compatibility issues.
Inorganic polymers such as hydroxyl aluminum and zirconium oxychloride are composed of multivalent metal ions that have the capability to hydrolyze in aqueous solutions to form polynuclear complexes with high positive charges. The high positive charges absorb into the negatively charged clay surface to stabilize clay minerals for a long period of time. Inorganic polymers are sensitive to pH and temperature ranges and their effectiveness changes depending on those conditions. The ultimate driver of inorganic polymers is their proven ability to treat clays for long period of time at a low cost and minimum HSE risks. Yet, their biggest disadvantage is their sensitivity to pH and temperature ranges.
Organic polymers include cationic organic polymers, anionic organic polymers, and nonionic organic polymers. Organic polymers have a relatively large molecular size which means they have the tendency to clog low-permeability formations with small pore sizes. However, when used in high-permeability formations they can provide long-term clay stabilization with relatively low HSE risks.
Acids are another form of clay stabilizer, useful in chlorite-rich formations. Acids can dissolve clay formations, preventing them from swelling or dispersing. There are two types of acids used for clay stabilization: single acids such as HF, HCl, and HBF4, and combined acids such as mud acids. The impact of acids is long term with low associated cost. However, their HSE risk are high with a potential to reduce the rock matrix strength.
Alkalis are another effective clay stabilizer that reduces swelling. Examples include NaOH and KOH. These stabilizers react with both silica and alumina sheets, which deactivates the clay’s water sensitivity and swelling capabilities. The reactions are a function of the concertation, temperature, and contact time. Benefits include long-term treatment of clay stabilization and a low relative cost. The biggest disadvantage of alkalis is a high HSE risk and low reaction rate at low temperatures.
Organic oligomers are yet another clay stabilizer. Unlike organic polymers, organic oligomers were developed to support clay stabilization in tight formations that have low permeabilities. One of the main advantages of organic oligomers is rooted in their ability to be effective even at low concentrations, hence lowering the overall operational cost. In addition, organic oligomers have low HSE risk. Their disadvantages are fast degradation and compatibility challenges with other in-situ fluids.
Nanoparticles have been also explored in the context of clay stabilization. Nanoparticles such as SiO2, Al2O3, and MgO were found through experimental studies to only mitigate for clay fine migrations and to be ineffective when it comes to mitigating formation damage due to clay swelling. The reason behind this assertion is that nanoparticles are small and therefore when exposed to the pore throats of a given formation, they might have tendencies to plug the pore spaces.
In summary, clay minerals are a vital component of many sedimentary formations. The presence of clay minerals in formations carries an associated risk of formation damage. Currently, there is a no one-size-fits-all formula for tackling clay induced formation damage.
However, there are many different clay stabilizers where each can be used under specific conditions. Research is ongoing to find a novel clay stabilizer that can provide longevity, sustainability, and can guarantee low HSE risks.
It is worth reminding also that this area of research is yet to mature. Understanding the market and its available resources is a powerful tool that aids in the future development of clay stabilizers and knowing which type to use given specific conditions.
For Further Reading
SPE 62524 Clay Stabilization Improves Sand Control by J.R. Tague, Chevron, and S. Lewallen, Halliburton.
Clay Stabilization in Sandstone Reservoirs and the Perspective of Shale Reservoirs by L. Wang, Nazarbayev University (Kazakhstan). Advances in Colloid and Interface Science.
SPE 21556 Laboratory Study Provides Guidelines for Selecting Clay Stabilizer by C.W. Crowe, Dowell Schlumberger.
SPE 132152 Maximizing Production Life With the Use of Nanotechnology To Prevent Fines Migration by C. Belcher, K. Seth, R. Hollier, Baker Hughes International, and Barney Paternostro, LLOG.
Novel Gemini Surfactant as a Clay-Stabilizing Additive in Fracturing Fluids for Unconventional Tight Sandstone: Mechanism and Performance by Z. Tariq, M.S. Kamal, and M. Mahmoud, et al., King Fahd University of Petroleum & Minerals. Journal of Petroleum Science and Engineering.