In this work, the authors demonstrate at a laboratory scale the possibility of using a radio-frequency heating system as a heat exchanger. The system consists of a pipe packed with nanoparticle-doped, superparamagnetic spheres as the bed material. This setup is able to heat in an efficient and temperature-accurate manner for a wide range of flow conditions, and can be potentially used as a highly efficient heat exchanger for production operations in areas where the construction of surface facilities is not possible.
Introduction
A porous bed, made of solid spheres doped with superparamagnetic material and inductively heated, was evaluated for its ability to increase the internal energy of flowing fluids. The power dissipation through this type of porous system is nominally constant axially and variable radially.
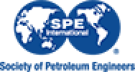