The Vega field on the Norwegian Continental Shelf has been producing successfully using continuous monoethylene glycol (MEG) injection, topped with means of corrosion inhibition. A topside reclamation process allows reuse of MEG but limits the possibilities of producing saline water. The complete paper presents a discussion of a feasibility study of a new flow-assurance and -integrity philosophy to manage wells without continuous MEG injection. The paper describes options for hydrate and integrity management and the required modifications to both subsea and topside facilities to enable an operational philosophy change.
Current Subsea Flow-Assurance Approaches
Gas-hydrate formation in wet gas flowlines is considered one of the primary challenges seen in subsea assets.
×
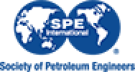
Continue Reading with SPE Membership
SPE Members: Please sign in at the top of the page for access to this member-exclusive content. If you are not a member and you find JPT content valuable, we encourage you to become a part of the SPE member community to gain full access.