A major issue with gamma-ray (GR) logs is that the API definition is valid only if the tool is run in a 4.89‑in. borehole filled with fresh water. Although all GR tools are intended to provide the same results for such a well, in the field there is no single one-size-fits-all concept. In this paper, the authors discuss the characterization process for GR tools and how they behave in boreholes different from the one used in the University of Houston (UH) GR characterization pit.
×
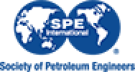
Continue Reading with SPE Membership
SPE Members: Please sign in at the top of the page for access to this member-exclusive content. If you are not a member and you find JPT content valuable, we encourage you to become a part of the SPE member community to gain full access.