The oil and gas industry faces a rapidly changing world of multiple challenges and opportunities, especially with growing climate and sustainability concerns. About a decade ago, SPE conducted a series of workshops to define the grand challenges in technology and R&D for the upstream oil and gas industry for the following decade. It identified 5 + 1 grand challenges (Table 1).
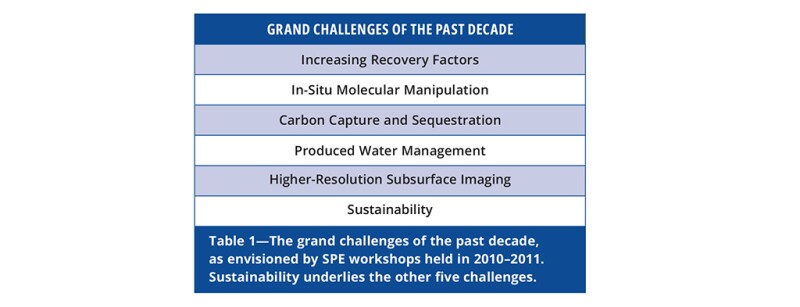
To define the set of grand challenges for the next decade, in a much more unpredictable environment, we held an SPE workshop in Austin, Texas, in January 2023. Panelists from IOCs, NOCs, and service companies, as well as environmental and financial stakeholders in the emerging energy system, surveyed the rapidly changing energy landscape. The workshop then facilitated sessions of the more than 120 thought leader participants to define grand challenges that are impactful for society and our industry and have a significant R&D/technology component that is aligned with the capabilities of the SPE membership.
We arrived at a list of five technical grand challenges: improved recovery from tight/shale resources, net-zero operations, carbon capture, utilization, and storage (CCUS), geothermal energy, and digital transformation. Additionally, we identified a sixth “bonus” nontechnical challenge—education and advocacy, since we believe that more effective engagement with thought leaders outside our industry will be required to advance and deploy new technologies in all five of these areas. Table 2 summarizes the technical challenges and their impacts.
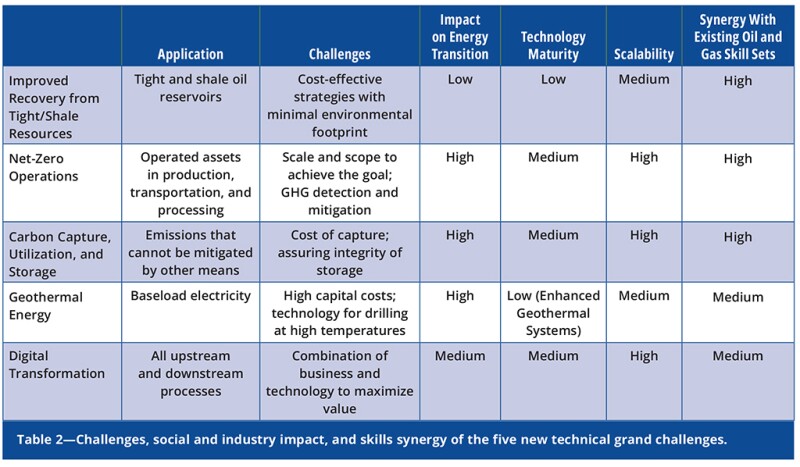
We believe that this work will help the industry focus its R&D and technology efforts on the highest-value activities for both industry and society. We now summarize each of these new grand challenges.
Improved Recovery From Tight/Shale Resources
Notwithstanding the rapid growth of non-hydrocarbon energy sources and carriers, oil and natural gas demand are projected to continue rising through 2050 (EIA 2023). In the past 20 years, horizontal drilling and hydraulic fracturing were combined to extract hydrocarbons from unconventional shale resources, which helped us meet rising global oil demand. However, production from existing shale oil wells declines quickly, and ultimate recoveries are often less than 10%. To meet global demand, we must improve recovery with minimal environmental impact.
Tight and shale oil reservoirs have unique challenges. For example, shale reservoirs are subject to clay swelling and formation damage. Permeabilities are ultra-low, often on the order of nano-Darcys, which leads to extremely low flow rates and to transport dominated by diffusion. Thus, hydraulic and natural fractures are necessary, and geomechanics/rock compaction plays an important role.
Many traditional improved oil recovery (IOR) strategies are challenging in tight and shale reservoirs. The small pores make waterflooding techniques difficult, and the low permeability hampers well-to-well methods. Thus, single-well, huff-and-puff strategies are the most common IOR methods. Injectants include miscible and immiscible gases (CO2, CH4, and N2) and chemicals (surfactants, solvents, and nanoparticles). In addition, thermal methods may allow control of oil viscosity and pressurization of the reservoir. Challenges with injectants include understanding their transport in the matrix, and their interaction with fractures, as well as finding the optimal injectants, pressures, soaking times, and cycle numbers in huff-and-puff operations. Additional benefits of gas huff-and-puff might include storage of greenhouse gases (CO2 and CH4).
Net-Zero Upstream Operations
The scale of the emissions reduction opportunity from oil and gas (O&G) operations (including abandoned wells) is significant. According to the IEA (2023), in 2020, scope 1 and 2 emissions from operations and purchased energy reached 5.3 Gt of CO2-equivalent (CO2-eq), or nearly 15% of global energy-derived greenhouse gas emissions.
Most of these emissions are attributed to the release of methane, venting CO2, and flaring. These emissions not only scale with O&G production but also depend on the asset (e.g., heavy oil). Emissions from extraction and product transport play a minor role. International shipping contributes a relatively small amount of emissions but represents an area of potential improvement.
Technologies to detect fugitive emissions exist today, so the technology roadmap to curb these emissions could be classified at a high Technology Readiness Level. But detection is only the first step. Significant effort will be required to upgrade existing infrastructure to minimize these emissions and apply processes to monitor them. A similar level of effort will be necessary to rethink energy consumption across all operations and implement renewable energy and low-carbon solutions wherever possible.
Addressing fugitive emissions and scaling existing and emerging low-carbon solutions requires a concerted effort across the industry and its value chain. However, the industry will have little credibility as a partner with society for addressing emissions concerns overall if it does not rapidly and aggressively address its own emissions.
Carbon Capture, Utilization, and Storage
Net global anthropogenic greenhouse gas emissions reached nearly 60 Gt CO2-eq in 2019, with energy-related emissions contributing the largest amount at nearly 40 Gt (IPCC 2022). CO2 is emitted from industrial sectors, transportation sectors, and from widely distributed residential and commercial buildings. Although wide-scale use of electrification and hydrogen are expected to address some of these emission sources, technology pathways to abate emissions from industrial sectors are challenging.
Carbon capture, identified as a critical technology by the IPCC, separates CO2 from gaseous streams emitted from numerous industrial processes. Two key categories of capture technology are post-combustion and pre-combustion capture, with a third emerging category of direct air capture. Post-combustion technologies include amine absorption, adsorption, and membrane separation. Pre-combustion technologies use pure oxygen in combustion. For broad commercial deployment, energy use and costs for CO2 capture need to be reduced, and options must be developed for permanent utilization or storage of CO2 at scale.
Captured CO2 can be stored in saline aquifers and depleted hydrocarbon reservoirs, requiring pressurization and transportation to geological storage sites. Aquifers need to be characterized for storage assurance. Hydrocarbon reservoirs are already characterized, but old wells need to be located and possibly sealed to assure permanent storage. The storage reservoirs need to be monitored for assurance and remediation. Monitoring technologies include pressure and seismic monitoring in wells, chemical monitoring in zones above the storage layer, and 4D seismic.
Today, regional supply chains linking CO2 capture sites, pipelines, and storage reservoirs are lacking. CCUS hubs can take advantage of economies of scale by combining resources and sharing CO2 transportation and storage among several emitters, thereby distributing the risk and costs of planning, implementing, and operating the full-chain CCUS process among several industries. For these solutions to succeed at scale, we will require new business models and new policies, including addressing long-term liability for CO2 sequestration.
Geothermal Energy
Geothermal energy offers the possibility of a low-carbon base load source of electric power that does not depend on the time of day or weather conditions. Large, high-enthalpy systems like The Geysers in California have provided gigawatts of power for decades. In 2022, the utilization of geothermal for electricity production was 6% in California and 9.4% in Nevada. Efforts to repurpose oil and gas wells for geothermal power are underway, in addition to second-generation greenfield projects to utilize hot, dry rocks.
There are synergies between production of hydrocarbons and production of hot water for energy. The basic drilling technologies that allow access to these resources are similar, although geothermal drilling costs are high, and both technology and tools are challenged by the hard rocks and extreme temperatures. Current commercial geothermal wells need to circulate large volumes of fluid due to the lower energy density relative to hydrocarbons.
Electricity generation projects require mapping of subsurface heat flow and natural fracture networks. Host rocks for geothermal systems commonly have low porosity and matrix permeability, thus the process requires fractures to achieve the necessary high production rates. Fluid flow is controlled by fracture networks, and heat is supplied to the fluid from the rock by convective heating of the fluid as it passes through the fracture network. In enhanced geothermal systems, bulk permeability is enhanced through stimulation or other means while understanding and mitigating the risk of seismicity. The enormous development of and learning from hydraulic fracturing in shale and tight oil and gas resources can be readily applied for geothermal systems.
Digital Transformation
Although commodity prices have recently given our industry some relief, in the last half of the previous decade it became apparent that business models in both the operating and the service sector were not robust in a low-price environment. This long-term challenge to our industry will rapidly become urgent the next time prices drop, as they will.
The rapid advance of digital technologies is a cliché of modern industrial life and offers a unique opportunity to fundamentally change the cost model of the oil and gas industry. For the past 2 decades, starting with the “Digital Oil Field” and maturing through concepts of “Digital Transformation,” the oil and gas industry has sought to leverage digital technology to enhance business performance. Along the way, there has been a subtle but definite shift from “digitalization,” the use of digital technology in existing processes, to “digital transformation,” the redefinition of those processes and innovation of new processes in ways enabled by new technology.
In the past decade, there has been rapid advancement in all facets of digital technology—hardware, software, sensors, automation, analytics, and how we interact with machines. Specific technologies in this area include cloud computing, AI and machine learning applied to large seismic data sets, business process automation, much more sophisticated optimization in upstream production and downstream processing, robotics, and the merger of modern IT and operational technologies to support dramatic operational changes such as widespread unattended facilities. Digital technologies can also offer safety improvements, such as rig floor personnel monitoring using radio-frequency ID tags.
There are many technical challenges in combining domain expertise, data science, new analytics, and new computing models, especially to support computationally intensive upstream workflows in seismic processing, interpretation, and reservoir modeling and simulation.
Education and Advocacy
The oil and gas industry has not kept pace with the accusations lodged against it. While the industry has amply provided for global energy security, we have failed to educate the general public regarding the engineering, scientific, and business challenges of meeting the world’s energy needs. In many countries, there has been a decline in enrollment in engineering and geology programs related to energy production. The public does not understand the contributions of oil and gas to base load electricity production, nor do they recognize the tremendous strides made in reducing our CO2 emissions by switching from coal to natural gas for power generation.
In short, we have not justified to society our future as an industry critical to energy production. The best R&D/technology strategy will still fail if we lack public support for pilot projects, capable scientists and engineers to conduct research or implement technology, or if opinion leaders ignore the outcomes of our R&D efforts.
We need to 1) educate the global public and opinion leaders about the need for and realities of energy production; 2) attract innovative new scientists and engineers to our industry; and 3) educate our own industry members regarding approaches to and elements of the energy transition and their role in it.
The next decade will be pivotal to our industry’s critical role in global energy security. Emotional voices driving out sound science-based policy decisions can erode and ultimately destroy the energy-based civilization built over the past century. The oil and gas industry must adapt with new technologies and honest and aggressive advocacy, countering the outdated understanding of oil and gas in the “All of the Above” energy mix.
Acknowledgments
We are grateful to Roland Horne, SPE, Silviu Livescu, SPE, Nirvasen Moonsamy, and Eliz Ozdemir for helpful comments and criticisms. We are especially grateful to Indira Saripally, SPE, for a thorough review of the draft.
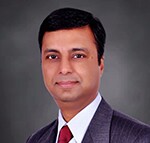
Gaurav Agrawal, SPE, is vice president, R&D at Newpark Resources. As the past chair of the SPE R&D Technical Section, he has organized events in energy transition, nanotechnology, and other areas. Previously he was vice president of the Saudi Arabia Dhahran Technology Center at Baker Hughes. He has over 80 granted US patents.
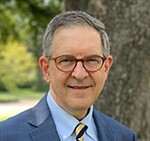
Jeffrey R. Bailey, ScD, SPE, worked at ExxonMobil for 32 years in drilling and subsurface technology. He retired as principal drilling mechanics engineer and has 28 publications and 32 patents. He was an SPE Distinguished Lecturer in 2020–2021 with a presentation on “Tuning a Drilling Assembly Like a Violin.”
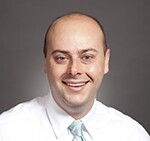
Matthew Balhoff, SPE, is a professor in the Hildebrand Department of Petroleum and Geosystems Engineering at UT-Austin. He holds a BS and PhD in chemical engineering from LSU. He became an SPE Distinguished Member in 2017 and received the 2022 SPE Lester C. Uren Award.
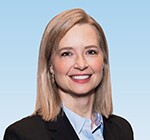
Scyller J. Borglum, PhD, SPE, has more than 15 years’ experience working in upstream, midstream, and adjacent energy industries including geomechanical laboratory research. She is currently vice president of energy and underground storage for WSP USA.
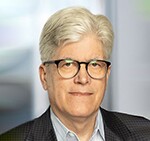
Thomas C. Halsey, SPE, retired in 2021 from ExxonMobil as chief computational scientist after a 26-year career. He is currently a lecturer in the Department of Chemical and Biomolecular Engineering at Rice University and is the chair of the SPE R&D Technical Section.
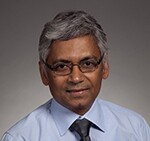
Kishore K. Mohanty, SPE, is the W.A. (Monty) Moncrief Centennial Chair Professor in Petroleum Engineering in the Hildebrand Department of Petroleum and Geosystems Engineering at UT-Austin. His research interests are in carbon capture and storage, enhanced oil recovery, and nanotechnology.
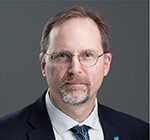
Michael Traver, PhD, SPE, currently works as a senior researcher in the Transport Technologies Division at Aramco Research Center–Detroit. He also chairs the Transportation Workstream at the Oil and Gas Climate Initiative, a voluntary, CEO-led oil and gas industry initiative to catalyze actions on climate change through collaboration and engagement.