In recent years, much progress has been made harnessing offshore renewable energy (ORE)—wind, wave, and current—for use in electrical supply. The complete paper is aimed at giving the newcomer to ORE, both with regard to wind and marine-hydrokinetic (MHK) devices, a basic understanding of the subject. The complete paper focuses on physical and technical issues and does not detail financial aspects of such projects.
Introduction
For any offshore development, especially an ORE project, a specific site investigation is required to qualify environmental, geophysical, metocean-related, and geopolitical issues. Most ORE developments will cover a significant area of ocean or seabed and will require investigation to ensure that marine life, antiquities, unexploded ordinance, and other ocean users will not be put at risk when installation and operation activities are performed.
Obtaining the required permits and approvals from all those potentially affected by an ORE development is a complex and time-consuming operation.
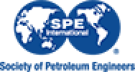