Corrosion inhibitors are often the first line of defense against internal corrosion, and effective mitigation relies on proactive monitoring and management of these inhibitors to allow for regular feedback and dose adjustment. There have been recent developments in this field, for example in greener chemistries (NACE 2016-7738) and treatment methods (Achour et al. 2008) and a growth in the number of papers published in OnePetro. We found an 18% increase in the number of publications in the last 10 years, compared with the previous 10, when searching the terms “corrosion AND inhibitor”.
Nevertheless, there is room for improvement. Common industry opinion is that current residual monitoring methods, such as colorimetric-complex methods or liquid chromatography mass spectrometry are either not sufficiently reliable for effective inhibitor dosage management or too complex to apply in the field.
Yet there is a significant commercial driver, as dosing corrosion inhibitors is an expensive undertaking and the potential cost improvements from improved management are significant. One North Sea operator reported that it believed it was overdosing inhibitor at an extra cost of £400,000 per year. An alternative corrosion inhibitor monitoring approach, first published in 2011 (NACE 11071), exploits the formation of corrosion inhibitor micelles and poses the questions:
- Does micelle detection have a place in the inhibitor qualification process?
- Can it help inform chemical management in the field?
Micelles—a Recap
The most common class of corrosion inhibitor used in the oilfield is amphiphilic surfactant molecules, which form a barrier on the pipe surface to protect from corrosion. Above a certain concentration, nanoscale aggregates called corrosion inhibitor micelles are formed. This point is called the Critical Micelle Concentration (CMC) and is specific to the chemical and unique physical conditions found in each system.
The CMC has been shown to be an important factor in establishing an effective inhibitor dose, with the optimum dose being equivalent to the CMC (e.g. NACE 10326). Below the CMC, there is an opportunity to dose additional inhibitor to further reduce corrosion while above the CMC, surplus inhibitor may be present.
Earlier studies looking at CMC and its relation to optimal inhibitor dose were relatively simplistic laboratory studies. Out of the 43 CMC studies reviewed, only one study used formulated inhibitors, with the majority looking only at single components, e.g. an imidazoline. Importantly, all 43 studies used model systems to test the corrosion inhibitors, rather than complex field fluids. It is therefore pertinent to address the question of whether micelles and the significance of the CMC are still relevant in real field conditions.
Inhibitor Qualification
Looking at corrosion inhibitor qualification, examples of best practice in the field can be found from the National Association of Corrosion Engineers (NACE) and the American Society for Testing Materials (ASTM) (ASTM G170 G184 G202 G185 G208; NACE Publication 1D196). There is no one test that can select the most appropriate inhibitor; a tailored approach for each system is required. Common qualification tests include static linear polarization resistance (LPR), rotating cylinder electrode, emulsion tests, and field trial.
Each test helps to form a picture of inhibitor suitability for each unique system. Static LPR tests, for example, are considered useful for ranking an inhibitor’s efficiency but not necessarily for identifying the best product or dosage for the field.
In a laboratory test designed to look at inhibitor efficiency and its link to micelle formation, LUX Assure conducted static LPR tests on five different commercial corrosion inhibitors using its corrosion inhibitor monitoring technology CoMic. Results showed that for only two of the five inhibitors tested, micelles, as detected by CoMic, were present at the point at which the corrosion rate stabilized. Given that these static tests offer a relatively unchallenging corrosive environment, conditions were then made slightly more challenging by introducing flow. Results found that for four of the same five inhibitors, micelles were present at the point at which the corrosion rate stabilized.
To make the testing yet more field-like, a multiphase flow-loop test was conducted (6 L of total fluids at a ratio of 80:20 [v/v] brine to oil, synthetic brine sparged with carbon dioxide and inhibitor free crude oil, from a North Sea field; flow rate of 10 L/min, and carbon dioxide pressure of 100 psi). Corrosion inhibitor dose was increased sequentially and micelle content of samples from the loop determined. Corrosion rate was measured using two LPR probes and a weight-loss coupon, which was changed for each dose.
A good correlation was observed between micelle formation and the point at which the corrosion rate stabilized. No tangible improvement in the general corrosion rate was observed when the corrosion inhibitor dose was >100 ppm, which was also the first dose at which micelles were detected (Figs. 1a and 1b).
Micelle Determination in Field
This technology will have most benefit in the field where simple on-site analysis technologies could provide considerable benefit to operators. Tests conducted at four field sites are reported here. All testing was done on site, using freshly taken samples (to minimize losses to containers and precipitates).
Micelles were detected in produced water from three of the four fields, (Table 1). Some assets were more suitable than others, with assets using continuously dosed, water–soluble, film-forming surfactant type chemicals being the most likely to benefit from micelle detection technology.
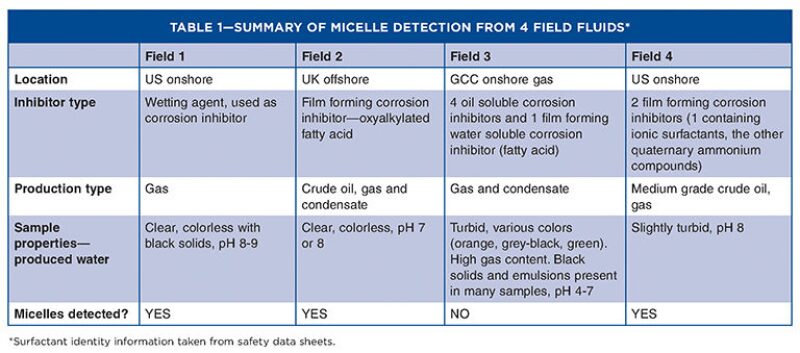
Dosage changes were made while on site at fields 1 and 2. For field 1, results showed that when the injection rate was reduced, micelles were no longer present in samples (Fig. 2a), and it was recommended that dosage remain at the original 110-ppm level.
For field 2, initially no micelles were detected in the samples, which alerted the operators to the fact that the inhibitor pump had been inadvertently switched off. Micelles were subsequently observed in samples analyzed, once the pump had been switched back on, (Fig. 2b).
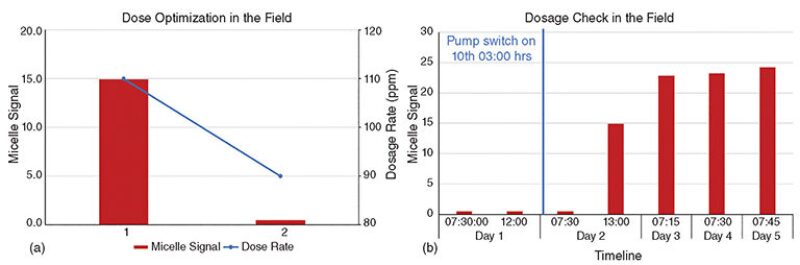
Conclusion
The concept of surfactant micelle formation is not new, nor is its application to the oilfield. However, data on this topic has previously been lacking. LUX Assure has shown that static LPR tests alone do not adequately represent field conditions, and the authors do not believe it is a valid technique for extrapolating dose to field systems.
For the first time, a link has been established between micelles and corrosion rate in more challenging tests, based on real field fluids and using commercially available corrosion inhibitors. The outcome of this has been the development of a credible technique for looking at the presence of micelles in field systems, which will contribute to better decision making.
References
Achour, M. et al. 2008. Comprehensive Laboratory Screening of Corrosion Inhibitors—A Key First Step in a Successful Corrosion Inhibition Treatment. Paper MECC 08094 presented at the Middle East Corrosion Conference, Bahrain, 3–6 February.
NACE 10326 Effect of Corrosion Inhibitor Active Components on Corrosion Inhibition in a Sweet Environment by K. Tsui et al. isbn:10326 2010 CP
NACE 11071 A Novel and Rapid System To Analyze Brines for the Optimum Functional Dose of Corrosion Inhibitor by C.D. Mackenzie et al. isbn:11071 2011 CP.
NACE-2016-7738 Development of a New Environmentally Compliant CO2 Corrosion Inhibitor for Subsea Injection by C.M. Menendez et al.