Reservoir simulation is valuable in understanding dynamics of unconventional reservoirs. Applications include estimating long-term production behavior, enhancing well-spacing and pad-modeling efficiency, optimizing completion and stimulation of horizontal wells, and understanding production drivers that cause differences in productivity between wells. In the complete paper, the authors revisit fundamental concepts of reservoir simulation in unconventional reservoirs and summarize several examples that form part of an archive of lessons learned.
Reservoir Simulation Applications in Unconventionals
Reservoir simulation plays an important role in many stages of unconventional reservoir field development. In the initial pilot phase, a calibrated reservoir simulation model can provide an indication of estimated ultimate recovery (EUR), which is advantageous given few wells with short production time.
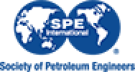