With the easy conventional oil in Argentina having been produced, one remaining way to find new oil in existing fields is to convert fields from primary or secondary production to secondary or tertiary production, respectively. For the Cerro Fortunoso field, the high costs required to develop the field, combined with an ambiguous log response that suggested that sand was predominantly disconnected, resulted in secondary production not being implemented. To reduce the risk, a waterflood pilot was necessary to demonstrate that waterflooding has potential and to provide water-injection and production data to constrain the history-matching process.
Introduction
Fig. 1 shows the location of Cerro Fortunoso in Mendoza province, approximately 1200 km southeast of Buenos Aires.
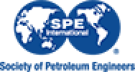